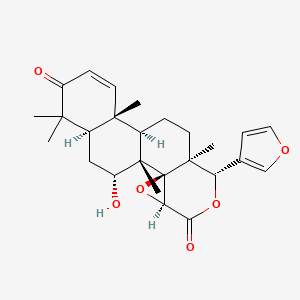
Deacetylgedunin
Overview
Description
Deacetylgedunin is a naturally occurring pentacyclic triterpenoid secondary metabolite found in the Meliaceae family. It is a derivative of gedunin and has been isolated from plants such as Azadirachta indica (neem) and Cedrela odorata . This compound has gained attention due to its potential bioactive properties, including anti-inflammatory, anti-cancer, and anti-malarial activities .
Preparation Methods
Synthetic Routes and Reaction Conditions: Deacetylgedunin can be synthesized through the deacetylation of gedunin. The process involves the removal of the acetyl group from gedunin using specific reagents and conditions. One common method is the use of basic hydrolysis, where gedunin is treated with a base such as sodium hydroxide (NaOH) in an aqueous or alcoholic medium .
Industrial Production Methods: Industrial production of this compound typically involves the extraction of gedunin from plant sources followed by its chemical modification. The extraction process includes solvent extraction using solvents like methanol or ethanol, followed by purification steps such as column chromatography .
Chemical Reactions Analysis
Types of Reactions: Deacetylgedunin undergoes various chemical reactions, including:
Oxidation: this compound can be oxidized to form different derivatives. For example, oxidation with reagents like potassium permanganate (KMnO4) can yield oxidized products.
Reduction: Reduction reactions can convert this compound into reduced forms using reducing agents like sodium borohydride (NaBH4).
Substitution: this compound can undergo substitution reactions where functional groups are replaced with other groups.
Common Reagents and Conditions:
Oxidation: Potassium permanganate (KMnO4), hydrogen peroxide (H2O2)
Reduction: Sodium borohydride (NaBH4), lithium aluminum hydride (LiAlH4)
Substitution: Halogenating agents like bromine (Br2), chlorine (Cl2)
Major Products Formed: The major products formed from these reactions depend on the specific reagents and conditions used. For example, oxidation of this compound can yield oxidized derivatives with different functional groups .
Scientific Research Applications
Chemistry: Deacetylgedunin serves as a valuable intermediate for the synthesis of other bioactive compounds.
Biology: this compound has shown promising anti-inflammatory and anti-cancer properties.
Medicine: this compound is being investigated for its potential as an anti-malarial agent.
Industry: this compound is used in the development of natural product-based pharmaceuticals and nutraceuticals.
Mechanism of Action
The mechanism of action of deacetylgedunin involves its interaction with specific molecular targets and pathways:
Anti-inflammatory Action: this compound activates the Keap1/Nrf2/HO-1 signaling pathway, leading to the suppression of inflammatory responses.
Anti-cancer Action: this compound induces cell cycle arrest and apoptosis in cancer cells.
Anti-malarial Action: this compound interferes with the life cycle of malaria parasites by inhibiting key enzymes and proteins essential for their survival.
Comparison with Similar Compounds
Deacetylgedunin is unique compared to other similar compounds due to its specific bioactive properties and molecular structure. Some similar compounds include:
Gedunin: The parent compound of this compound, known for its anti-malarial and anti-cancer properties.
Deacetoxy-7-oxogedunin: Another derivative of gedunin with similar bioactive properties.
Deoxygedunin: A derivative with potential neuroprotective effects.
This compound stands out due to its specific anti-inflammatory and anti-cancer activities, making it a valuable compound for further research and development.
Properties
IUPAC Name |
(1S,2R,4S,7S,8S,11R,12R,17R,19R)-7-(furan-3-yl)-19-hydroxy-1,8,12,16,16-pentamethyl-3,6-dioxapentacyclo[9.8.0.02,4.02,8.012,17]nonadec-13-ene-5,15-dione | |
---|---|---|
Source | PubChem | |
URL | https://pubchem.ncbi.nlm.nih.gov | |
Description | Data deposited in or computed by PubChem | |
InChI |
InChI=1S/C26H32O6/c1-22(2)16-12-18(28)25(5)15(23(16,3)9-7-17(22)27)6-10-24(4)19(14-8-11-30-13-14)31-21(29)20-26(24,25)32-20/h7-9,11,13,15-16,18-20,28H,6,10,12H2,1-5H3/t15-,16+,18-,19+,20-,23-,24+,25+,26-/m1/s1 | |
Source | PubChem | |
URL | https://pubchem.ncbi.nlm.nih.gov | |
Description | Data deposited in or computed by PubChem | |
InChI Key |
HCEYJYMNIQHPPK-DXTZDJJUSA-N | |
Source | PubChem | |
URL | https://pubchem.ncbi.nlm.nih.gov | |
Description | Data deposited in or computed by PubChem | |
Canonical SMILES |
CC1(C2CC(C3(C(C2(C=CC1=O)C)CCC4(C35C(O5)C(=O)OC4C6=COC=C6)C)C)O)C | |
Source | PubChem | |
URL | https://pubchem.ncbi.nlm.nih.gov | |
Description | Data deposited in or computed by PubChem | |
Isomeric SMILES |
C[C@@]12CC[C@@H]3[C@]4(C=CC(=O)C([C@@H]4C[C@H]([C@]3([C@@]15[C@H](O5)C(=O)O[C@H]2C6=COC=C6)C)O)(C)C)C | |
Source | PubChem | |
URL | https://pubchem.ncbi.nlm.nih.gov | |
Description | Data deposited in or computed by PubChem | |
Molecular Formula |
C26H32O6 | |
Source | PubChem | |
URL | https://pubchem.ncbi.nlm.nih.gov | |
Description | Data deposited in or computed by PubChem | |
Molecular Weight |
440.5 g/mol | |
Source | PubChem | |
URL | https://pubchem.ncbi.nlm.nih.gov | |
Description | Data deposited in or computed by PubChem | |
CAS No. |
10314-90-6 | |
Record name | Deacetylgedunin | |
Source | CAS Common Chemistry | |
URL | https://commonchemistry.cas.org/detail?cas_rn=10314-90-6 | |
Description | CAS Common Chemistry is an open community resource for accessing chemical information. Nearly 500,000 chemical substances from CAS REGISTRY cover areas of community interest, including common and frequently regulated chemicals, and those relevant to high school and undergraduate chemistry classes. This chemical information, curated by our expert scientists, is provided in alignment with our mission as a division of the American Chemical Society. | |
Explanation | The data from CAS Common Chemistry is provided under a CC-BY-NC 4.0 license, unless otherwise stated. | |
Record name | Deacetylgedunin | |
Source | ChemIDplus | |
URL | https://pubchem.ncbi.nlm.nih.gov/substance/?source=chemidplus&sourceid=0010314906 | |
Description | ChemIDplus is a free, web search system that provides access to the structure and nomenclature authority files used for the identification of chemical substances cited in National Library of Medicine (NLM) databases, including the TOXNET system. | |
Retrosynthesis Analysis
AI-Powered Synthesis Planning: Our tool employs the Template_relevance Pistachio, Template_relevance Bkms_metabolic, Template_relevance Pistachio_ringbreaker, Template_relevance Reaxys, Template_relevance Reaxys_biocatalysis model, leveraging a vast database of chemical reactions to predict feasible synthetic routes.
One-Step Synthesis Focus: Specifically designed for one-step synthesis, it provides concise and direct routes for your target compounds, streamlining the synthesis process.
Accurate Predictions: Utilizing the extensive PISTACHIO, BKMS_METABOLIC, PISTACHIO_RINGBREAKER, REAXYS, REAXYS_BIOCATALYSIS database, our tool offers high-accuracy predictions, reflecting the latest in chemical research and data.
Strategy Settings
Precursor scoring | Relevance Heuristic |
---|---|
Min. plausibility | 0.01 |
Model | Template_relevance |
Template Set | Pistachio/Bkms_metabolic/Pistachio_ringbreaker/Reaxys/Reaxys_biocatalysis |
Top-N result to add to graph | 6 |
Feasible Synthetic Routes
Disclaimer and Information on In-Vitro Research Products
Please be aware that all articles and product information presented on BenchChem are intended solely for informational purposes. The products available for purchase on BenchChem are specifically designed for in-vitro studies, which are conducted outside of living organisms. In-vitro studies, derived from the Latin term "in glass," involve experiments performed in controlled laboratory settings using cells or tissues. It is important to note that these products are not categorized as medicines or drugs, and they have not received approval from the FDA for the prevention, treatment, or cure of any medical condition, ailment, or disease. We must emphasize that any form of bodily introduction of these products into humans or animals is strictly prohibited by law. It is essential to adhere to these guidelines to ensure compliance with legal and ethical standards in research and experimentation.