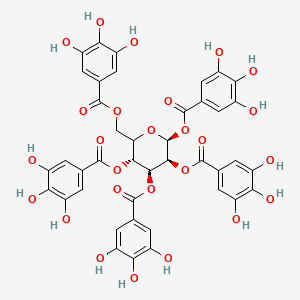
Pentagalloylglucose
Overview
Description
Pentagalloylglucose is a natural hydrolyzable gallotannin, which is the pentagallic acid ester of glucose. It is found in various plants and herbs, including pomegranate, mango, and tree peony . This compound has garnered significant attention due to its broad range of biological activities, including antimicrobial, anti-inflammatory, anticarcinogenic, antidiabetic, and antioxidant properties .
Mechanism of Action
Pentagalloylglucose (PGG) is a natural hydrolyzable gallotannin abundant in various plants and herbs, known for its broad range of biological activities .
Mode of Action
PGG interacts with its targets to inhibit their function. For instance, it effectively blocks ACE in a direct chemical reaction . It also acts on multiple molecular targets and signaling pathways associated with cancer to inhibit growth, angiogenesis, and metastasis . Moreover, PGG can enhance the efficacy of chemotherapy and radiotherapy by modulating various cancer-associated pathways .
Biochemical Pathways
PGG affects several biochemical pathways. It acts on signaling pathways associated with the hallmarks of cancer, inhibiting growth, angiogenesis, and metastasis of several cancers . It also modulates various cancer-associated pathways to enhance the efficacy of chemotherapy and radiotherapy .
Pharmacokinetics
The data on the pharmacokinetics of PGG are limited . It’s known that pgg has significant biomedical benefits, such as being a free radical sink, an anti-inflammatory agent, anti-diabetic agent, and having enzymatic resistant properties .
Result of Action
The molecular and cellular effects of PGG’s action are diverse. It inhibits the growth, angiogenesis, and metastasis of several cancers . It also maintains the aortic elastin architecture, tempers the aneurysm diameter expansion, and prevents aneurysm development .
Action Environment
The action, efficacy, and stability of PGG can be influenced by environmental factors. For instance, the extraction solvent used for PGG can affect its properties . Ethanol or methanol are commonly used, but water and aqueous acetone have also been used .
Biochemical Analysis
Biochemical Properties
PGG has been found to interact with a variety of enzymes, proteins, and other biomolecules. For instance, it can precipitate proteins, including human salivary α-amylase . It also has the ability to interfere with lipid layers and strongly inhibit the activity of a variety of enzymes . These interactions are largely due to the presence of multiple galloyl groups in the PGG molecule, which can form hydrogen bonds and hydrophobic interactions with other biomolecules .
Cellular Effects
PGG has been shown to have significant effects on various types of cells and cellular processes. It acts on multiple molecular targets and signaling pathways associated with the hallmarks of cancer to inhibit growth, angiogenesis, and metastasis of several cancers . Moreover, PGG can enhance the efficacy of chemotherapy and radiotherapy by modulating various cancer-associated pathways .
Molecular Mechanism
The molecular mechanism of action of PGG involves its interactions with various biomolecules at the molecular level. For example, it can bind to proteins and precipitate them, which can disrupt their normal function . Additionally, PGG can inhibit the activity of various enzymes, potentially altering cellular metabolic pathways .
Temporal Effects in Laboratory Settings
The effects of PGG can change over time in laboratory settings. For instance, PGG can undergo oxidation reactions depending on the pH
Metabolic Pathways
PGG is involved in various metabolic pathways. The enzyme beta-glucogallin-tetrakisgalloylglucose O-galloyltransferase uses 1-O-galloyl-beta-D-glucose and 1,2,3,6-tetrakis-O-galloyl-beta-D-glucose to produce D-glucose and pentagalloyl glucose .
Preparation Methods
Pentagalloylglucose can be synthesized through several methods. One common synthetic route involves the use of tannic acid. The preparation involves acidic methanolysis of tannic acid, yielding this compound with a typical yield of 15% . Industrial production methods often utilize ethanol or methanol as extraction solvents, although water and aqueous acetone have also been used .
Chemical Reactions Analysis
Pentagalloylglucose undergoes various chemical reactions, including oxidation, reduction, and substitution. The oxidation reactions of this compound are pH-dependent . Common reagents used in these reactions include acidic and basic solutions, as well as specific enzymes. Major products formed from these reactions include tellimagrandin II, which is formed by oxidative dehydrogenation and coupling of two galloyl groups .
Scientific Research Applications
Pentagalloylglucose has numerous scientific research applications across various fields:
Comparison with Similar Compounds
Pentagalloylglucose is unique due to its structure, which consists of five galloyl groups attached to a glucose core. Similar compounds include other gallotannins and ellagitannins, such as tellimagrandin II and hexa-galloylglucose . These compounds share some biological activities with this compound but differ in their specific molecular targets and pathways.
This compound stands out due to its high biological activity and potential therapeutic applications, making it a valuable compound for further research and development.
Properties
IUPAC Name |
[(2R,3R,4S,5R,6S)-3,4,5,6-tetrakis[(3,4,5-trihydroxybenzoyl)oxy]oxan-2-yl]methyl 3,4,5-trihydroxybenzoate | |
---|---|---|
Source | PubChem | |
URL | https://pubchem.ncbi.nlm.nih.gov | |
Description | Data deposited in or computed by PubChem | |
InChI |
InChI=1S/C41H32O26/c42-17-1-12(2-18(43)28(17)52)36(57)62-11-27-33(64-37(58)13-3-19(44)29(53)20(45)4-13)34(65-38(59)14-5-21(46)30(54)22(47)6-14)35(66-39(60)15-7-23(48)31(55)24(49)8-15)41(63-27)67-40(61)16-9-25(50)32(56)26(51)10-16/h1-10,27,33-35,41-56H,11H2/t27-,33-,34+,35-,41+/m1/s1 | |
Source | PubChem | |
URL | https://pubchem.ncbi.nlm.nih.gov | |
Description | Data deposited in or computed by PubChem | |
InChI Key |
QJYNZEYHSMRWBK-NIKIMHBISA-N | |
Source | PubChem | |
URL | https://pubchem.ncbi.nlm.nih.gov | |
Description | Data deposited in or computed by PubChem | |
Canonical SMILES |
C1=C(C=C(C(=C1O)O)O)C(=O)OCC2C(C(C(C(O2)OC(=O)C3=CC(=C(C(=C3)O)O)O)OC(=O)C4=CC(=C(C(=C4)O)O)O)OC(=O)C5=CC(=C(C(=C5)O)O)O)OC(=O)C6=CC(=C(C(=C6)O)O)O | |
Source | PubChem | |
URL | https://pubchem.ncbi.nlm.nih.gov | |
Description | Data deposited in or computed by PubChem | |
Isomeric SMILES |
C1=C(C=C(C(=C1O)O)O)C(=O)OC[C@@H]2[C@H]([C@@H]([C@H]([C@@H](O2)OC(=O)C3=CC(=C(C(=C3)O)O)O)OC(=O)C4=CC(=C(C(=C4)O)O)O)OC(=O)C5=CC(=C(C(=C5)O)O)O)OC(=O)C6=CC(=C(C(=C6)O)O)O | |
Source | PubChem | |
URL | https://pubchem.ncbi.nlm.nih.gov | |
Description | Data deposited in or computed by PubChem | |
Molecular Formula |
C41H32O26 | |
Source | PubChem | |
URL | https://pubchem.ncbi.nlm.nih.gov | |
Description | Data deposited in or computed by PubChem | |
DSSTOX Substance ID |
DTXSID901029765 | |
Record name | 1,2,3,4,6-Pentagalloyl glucose | |
Source | EPA DSSTox | |
URL | https://comptox.epa.gov/dashboard/DTXSID901029765 | |
Description | DSSTox provides a high quality public chemistry resource for supporting improved predictive toxicology. | |
Molecular Weight |
940.7 g/mol | |
Source | PubChem | |
URL | https://pubchem.ncbi.nlm.nih.gov | |
Description | Data deposited in or computed by PubChem | |
CAS No. |
14937-32-7 | |
Record name | Penta-O-galloyl-β-D-glucose | |
Source | CAS Common Chemistry | |
URL | https://commonchemistry.cas.org/detail?cas_rn=14937-32-7 | |
Description | CAS Common Chemistry is an open community resource for accessing chemical information. Nearly 500,000 chemical substances from CAS REGISTRY cover areas of community interest, including common and frequently regulated chemicals, and those relevant to high school and undergraduate chemistry classes. This chemical information, curated by our expert scientists, is provided in alignment with our mission as a division of the American Chemical Society. | |
Explanation | The data from CAS Common Chemistry is provided under a CC-BY-NC 4.0 license, unless otherwise stated. | |
Record name | Pentagalloylglucose | |
Source | ChemIDplus | |
URL | https://pubchem.ncbi.nlm.nih.gov/substance/?source=chemidplus&sourceid=0014937327 | |
Description | ChemIDplus is a free, web search system that provides access to the structure and nomenclature authority files used for the identification of chemical substances cited in National Library of Medicine (NLM) databases, including the TOXNET system. | |
Record name | 1,2,3,4,6-Pentagalloyl glucose | |
Source | EPA DSSTox | |
URL | https://comptox.epa.gov/dashboard/DTXSID901029765 | |
Description | DSSTox provides a high quality public chemistry resource for supporting improved predictive toxicology. | |
Record name | PENTAGALLOYLGLUCOSE | |
Source | FDA Global Substance Registration System (GSRS) | |
URL | https://gsrs.ncats.nih.gov/ginas/app/beta/substances/3UI3K8W93I | |
Description | The FDA Global Substance Registration System (GSRS) enables the efficient and accurate exchange of information on what substances are in regulated products. Instead of relying on names, which vary across regulatory domains, countries, and regions, the GSRS knowledge base makes it possible for substances to be defined by standardized, scientific descriptions. | |
Explanation | Unless otherwise noted, the contents of the FDA website (www.fda.gov), both text and graphics, are not copyrighted. They are in the public domain and may be republished, reprinted and otherwise used freely by anyone without the need to obtain permission from FDA. Credit to the U.S. Food and Drug Administration as the source is appreciated but not required. | |
Disclaimer and Information on In-Vitro Research Products
Please be aware that all articles and product information presented on BenchChem are intended solely for informational purposes. The products available for purchase on BenchChem are specifically designed for in-vitro studies, which are conducted outside of living organisms. In-vitro studies, derived from the Latin term "in glass," involve experiments performed in controlled laboratory settings using cells or tissues. It is important to note that these products are not categorized as medicines or drugs, and they have not received approval from the FDA for the prevention, treatment, or cure of any medical condition, ailment, or disease. We must emphasize that any form of bodily introduction of these products into humans or animals is strictly prohibited by law. It is essential to adhere to these guidelines to ensure compliance with legal and ethical standards in research and experimentation.