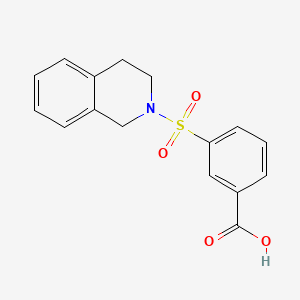
3-(3,4-dihydroisoquinolin-2(1H)-ylsulfonyl)benzoic acid
Overview
Description
AKR1C3-IN-1 is a potent and highly selective inhibitor of the enzyme aldo-keto reductase family 1 member C3 (AKR1C3). This enzyme plays a crucial role in the metabolism of steroids, including the conversion of weak androgens to potent androgens, and is implicated in various cancers, particularly prostate cancer .
Mechanism of Action
Target of Action
The primary target of 3-((3,4-Dihydroisoquinolin-2(1H)-yl)sulfonyl)benzoic acid, also known as AKR1C3-IN-1, is the aldo-keto reductase AKR1C3 . This enzyme is of interest in both breast and prostate cancer .
Mode of Action
AKR1C3-IN-1 is a highly potent and isoform-selective inhibitor of AKR1C3 . Crystal structure studies have shown that the carboxylate group of the compound occupies the oxyanion hole in the enzyme, while the sulfonamide provides the correct twist to allow the dihydroisoquinoline to bind in an adjacent hydrophobic pocket .
Biochemical Pathways
The inhibition of AKR1C3 by AKR1C3-IN-1 affects the metabolism of a known dinitrobenzamide substrate
Biochemical Analysis
Biochemical Properties
The compound interacts with the enzyme AKR1C3, which is part of the aldo/keto reductase superfamily . These enzymes catalyze the conversion of aldehydes and ketones to their corresponding alcohols by utilizing NADH and/or NADPH as cofactors . AKR1C3, in particular, has been found to have the highest catalytic efficiency in converting delta4-androstenedione to testosterone .
Cellular Effects
3-((3,4-Dihydroisoquinolin-2(1H)-yl)sulfonyl)benzoic acid has been shown to have significant effects on cancer cells. Elevated levels of AKR1C3, which this compound inhibits, have been correlated with increased cancer cell growth, proliferation, migration, and metastasis . Inhibiting AKR1C3 with this compound has proven effective in halting carcinoma progression .
Molecular Mechanism
The molecular mechanism of action of 3-((3,4-Dihydroisoquinolin-2(1H)-yl)sulfonyl)benzoic acid involves its interaction with the enzyme AKR1C3. Crystal structure studies have shown that the carboxylate group of the compound occupies the oxyanion hole in the enzyme, while the sulfonamide provides the correct twist to allow the dihydroisoquinoline to bind in an adjacent hydrophobic pocket .
Preparation Methods
Synthetic Routes and Reaction Conditions
The synthesis of AKR1C3-IN-1 involves several steps, including the preparation of intermediate compounds and their subsequent reactions. The synthetic route typically starts with the preparation of a core structure, followed by functional group modifications to enhance selectivity and potency. Common reagents used in these reactions include organic solvents like dimethyl sulfoxide (DMSO), catalysts, and various acids and bases .
Industrial Production Methods
Industrial production of AKR1C3-IN-1 involves large-scale synthesis using optimized reaction conditions to ensure high yield and purity. The process includes the use of automated reactors, continuous flow systems, and stringent quality control measures to maintain consistency and efficiency .
Chemical Reactions Analysis
Types of Reactions
AKR1C3-IN-1 undergoes several types of chemical reactions, including:
Oxidation: Conversion of alcohols to ketones or aldehydes.
Reduction: Conversion of ketones or aldehydes to alcohols.
Substitution: Replacement of one functional group with another.
Common Reagents and Conditions
Common reagents used in these reactions include oxidizing agents like potassium permanganate, reducing agents like sodium borohydride, and various acids and bases. Reaction conditions typically involve controlled temperatures, pH levels, and solvent systems to ensure optimal reaction rates and product formation .
Major Products Formed
The major products formed from these reactions include various steroid derivatives, which are crucial for studying the enzyme’s role in steroid metabolism and its implications in cancer .
Scientific Research Applications
AKR1C3-IN-1 has several scientific research applications, including:
Chemistry: Used as a tool compound to study the enzyme’s role in steroid metabolism.
Biology: Helps in understanding the enzyme’s function in various biological processes.
Medicine: Investigated for its potential in treating cancers, particularly prostate cancer, by inhibiting the enzyme’s activity.
Industry: Used in the development of new therapeutic agents targeting AKR1C3.
Comparison with Similar Compounds
AKR1C3-IN-1 is compared with other similar compounds, such as Stylopine and various indomethacin analogues. These compounds also inhibit AKR1C3 but differ in their selectivity and potency. AKR1C3-IN-1 is unique due to its high selectivity and potency, making it a promising candidate for therapeutic development .
List of Similar Compounds
- Stylopine
- Indomethacin analogues
- Non-steroidal anti-inflammatory drugs (NSAIDs)
- Flavonoids
- Cyclopentanes
Properties
IUPAC Name |
3-(3,4-dihydro-1H-isoquinolin-2-ylsulfonyl)benzoic acid | |
---|---|---|
Source | PubChem | |
URL | https://pubchem.ncbi.nlm.nih.gov | |
Description | Data deposited in or computed by PubChem | |
InChI |
InChI=1S/C16H15NO4S/c18-16(19)13-6-3-7-15(10-13)22(20,21)17-9-8-12-4-1-2-5-14(12)11-17/h1-7,10H,8-9,11H2,(H,18,19) | |
Source | PubChem | |
URL | https://pubchem.ncbi.nlm.nih.gov | |
Description | Data deposited in or computed by PubChem | |
InChI Key |
ZGVIUMKHTXKKOX-UHFFFAOYSA-N | |
Source | PubChem | |
URL | https://pubchem.ncbi.nlm.nih.gov | |
Description | Data deposited in or computed by PubChem | |
Canonical SMILES |
C1CN(CC2=CC=CC=C21)S(=O)(=O)C3=CC=CC(=C3)C(=O)O | |
Source | PubChem | |
URL | https://pubchem.ncbi.nlm.nih.gov | |
Description | Data deposited in or computed by PubChem | |
Molecular Formula |
C16H15NO4S | |
Source | PubChem | |
URL | https://pubchem.ncbi.nlm.nih.gov | |
Description | Data deposited in or computed by PubChem | |
DSSTOX Substance ID |
DTXSID20351900 | |
Record name | 3-(3,4-Dihydro-1H-isoquinoline-2-sulfonyl)-benzoic acid | |
Source | EPA DSSTox | |
URL | https://comptox.epa.gov/dashboard/DTXSID20351900 | |
Description | DSSTox provides a high quality public chemistry resource for supporting improved predictive toxicology. | |
Molecular Weight |
317.4 g/mol | |
Source | PubChem | |
URL | https://pubchem.ncbi.nlm.nih.gov | |
Description | Data deposited in or computed by PubChem | |
Solubility |
26.2 [ug/mL] (The mean of the results at pH 7.4) | |
Record name | SID49643309 | |
Source | Burnham Center for Chemical Genomics | |
URL | https://pubchem.ncbi.nlm.nih.gov/bioassay/1996#section=Data-Table | |
Description | Aqueous solubility in buffer at pH 7.4 | |
CAS No. |
327092-81-9 | |
Record name | 3-(3,4-Dihydro-1H-isoquinoline-2-sulfonyl)-benzoic acid | |
Source | EPA DSSTox | |
URL | https://comptox.epa.gov/dashboard/DTXSID20351900 | |
Description | DSSTox provides a high quality public chemistry resource for supporting improved predictive toxicology. | |
Retrosynthesis Analysis
AI-Powered Synthesis Planning: Our tool employs the Template_relevance Pistachio, Template_relevance Bkms_metabolic, Template_relevance Pistachio_ringbreaker, Template_relevance Reaxys, Template_relevance Reaxys_biocatalysis model, leveraging a vast database of chemical reactions to predict feasible synthetic routes.
One-Step Synthesis Focus: Specifically designed for one-step synthesis, it provides concise and direct routes for your target compounds, streamlining the synthesis process.
Accurate Predictions: Utilizing the extensive PISTACHIO, BKMS_METABOLIC, PISTACHIO_RINGBREAKER, REAXYS, REAXYS_BIOCATALYSIS database, our tool offers high-accuracy predictions, reflecting the latest in chemical research and data.
Strategy Settings
Precursor scoring | Relevance Heuristic |
---|---|
Min. plausibility | 0.01 |
Model | Template_relevance |
Template Set | Pistachio/Bkms_metabolic/Pistachio_ringbreaker/Reaxys/Reaxys_biocatalysis |
Top-N result to add to graph | 6 |
Feasible Synthetic Routes
Q1: What makes 3-(3,4-dihydroisoquinolin-2(1H)-ylsulfonyl)benzoic acid a promising lead compound for AKR1C3 inhibition?
A1: This compound exhibits potent inhibitory activity against AKR1C3 in the low nanomolar range []. Importantly, it displays high selectivity for AKR1C3, demonstrating a 1500-fold preference over other isoforms []. This selectivity makes it an attractive starting point for drug development, as it could minimize potential side effects arising from off-target interactions.
Q2: How does this compound interact with AKR1C3 at the molecular level?
A2: Crystal structure studies have revealed the specific interactions responsible for its inhibitory activity []. The carboxylate group of the compound occupies the oxyanion hole within the enzyme's active site. Simultaneously, the sulfonamide moiety adopts a specific conformation that allows the dihydroisoquinoline ring to bind within an adjacent hydrophobic pocket []. This dual-site binding contributes significantly to the compound's potency and selectivity.
Q3: What structural modifications of this compound were explored, and how did they impact its inhibitory activity?
A3: Structure-activity relationship (SAR) studies investigated various modifications to the parent compound []. These studies highlighted the importance of the carboxylate group's position for potent inhibition, although bioisosteric replacements like acid isosteres and amides were tolerated []. Interestingly, incorporating small substituents on the dihydroisoquinoline ring further enhanced inhibitory potency []. A series of "reverse sulfonamides" revealed a 12-fold preference for the R stereoisomer in terms of activity []. These findings provide valuable insights for optimizing this class of inhibitors for improved potency and selectivity.
Q4: Beyond direct enzyme inhibition, did this compound demonstrate cellular activity?
A4: Yes, the compound effectively inhibited AKR1C3 activity in a cellular context. Researchers measured this inhibition by monitoring the compound's ability to block AKR1C3-mediated metabolism of a known dinitrobenzamide substrate []. While a general correlation existed between enzyme and cellular activity, amide analogs surprisingly displayed greater efficacy in the cellular assay compared to their predicted activity based solely on enzyme inhibition data []. This discrepancy underscores the importance of evaluating compounds in cellular systems to gain a more comprehensive understanding of their overall efficacy and potential for therapeutic development.
Q5: Why is understanding the induction of AKR1C1 relevant in the context of PAH metabolism and carcinogenesis?
A5: Research indicates that exposure to polycyclic aromatic hydrocarbons (PAHs) can lead to the induction of AKR1C1 []. This enzyme converts PAH trans-dihydrodiols (proximate carcinogens) into reactive and redox-active ortho-quinones []. The concern arises because these ortho-quinones can generate reactive oxygen species (ROS), which have been implicated in both tumor initiation and promotion []. Therefore, the induction of AKR1C1 by PAHs could potentially create a vicious cycle, amplifying ROS production and thereby contributing to PAH carcinogenesis [].
Disclaimer and Information on In-Vitro Research Products
Please be aware that all articles and product information presented on BenchChem are intended solely for informational purposes. The products available for purchase on BenchChem are specifically designed for in-vitro studies, which are conducted outside of living organisms. In-vitro studies, derived from the Latin term "in glass," involve experiments performed in controlled laboratory settings using cells or tissues. It is important to note that these products are not categorized as medicines or drugs, and they have not received approval from the FDA for the prevention, treatment, or cure of any medical condition, ailment, or disease. We must emphasize that any form of bodily introduction of these products into humans or animals is strictly prohibited by law. It is essential to adhere to these guidelines to ensure compliance with legal and ethical standards in research and experimentation.