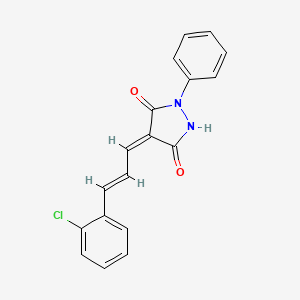
Cpypp
Overview
Description
Mechanism of Action
Target of Action
CPYPP is a DOCK2-Rac1 interaction inhibitor . It binds to the DOCK2 DHR-2 domain and inhibits the guanine nucleotide exchange factor (GEF) activity of DOCK2DHR-2 for Rac1 . In addition to DOCK2, this compound also inhibits DOCK180 and DOCK5, but less inhibits DOCK9 .
Mode of Action
This compound binds to the DOCK2 DHR-2 domain in a reversible manner and inhibits its catalytic activity . This results in the blockage of both chemokine receptor- and antigen receptor-mediated Rac activation , leading to a marked reduction of chemotactic response and T cell activation .
Biochemical Pathways
The primary biochemical pathway affected by this compound is the DOCK2-Rac1 signaling pathway . By inhibiting the interaction between DOCK2 and Rac1, this compound disrupts the normal functioning of this pathway, which plays a crucial role in cell migration and immune response .
Pharmacokinetics
It is known that when administered intravenously at a dose of 25 mg/kg, the plasma concentration of this compound is only 24 μM at 30 minutes . By contrast, intraperitoneal injection of 250 mg/kg of this compound into mice results in a plasma concentration of 11.3 μM at 30 minutes and 10.9 μM at 1 hour .
Result of Action
This compound has been shown to have significant effects at the molecular and cellular levels. It effectively decreases the secretion and gene expression of TNF-α, IL-1β, and IL-6 in the lungs, suggesting effects of DOCK2 on endotoxemia-induced inflammatory responses in mice . This compound also remarkably inhibits the infiltration of total cells, macrophages, and neutrophils into the bronchoalveolar lavage fluid .
Action Environment
The action of this compound can be influenced by various environmental factors. For instance, the concentration of this compound in the plasma can vary depending on the method of administration . .
Biochemical Analysis
Biochemical Properties
CPYPP plays a significant role in biochemical reactions by inhibiting the guanine nucleotide exchange factor (GEF) activity of DOCK2, DOCK5, and DOCK180 . It selectively inhibits these DOCK-A subfamily proteins over the DOCK-D subfamily member DOCK9 . This compound prevents chemokine-induced Rac activation in T and B cells, thereby inhibiting their migration . This inhibition is crucial for understanding the compound’s potential in modulating immune responses and inflammatory processes.
Cellular Effects
This compound has profound effects on various types of cells and cellular processes. In T and B cells, this compound inhibits chemokine-induced migration by preventing Rac activation . This inhibition affects cell signaling pathways, gene expression, and cellular metabolism. For instance, this compound reduces myeloperoxidase (MPO) activity in lung tissue and decreases cytokine release in serum, which are indicative of its anti-inflammatory properties . Additionally, this compound has been shown to reduce the severity of lung injury in a mouse model of endotoxemia-induced acute lung injury .
Molecular Mechanism
At the molecular level, this compound exerts its effects by binding to the DHR-2 domain of DOCK2, thereby inhibiting its GEF activity for Rac1 . This binding interaction prevents the activation of Rac1, a small GTPase involved in various cellular processes such as cell migration, proliferation, and survival . By inhibiting Rac1 activation, this compound disrupts downstream signaling pathways that are crucial for cell movement and immune responses.
Temporal Effects in Laboratory Settings
In laboratory settings, the effects of this compound have been observed to change over time. This compound is stable under standard storage conditions and has a shelf life of at least four years . In vitro studies have shown that this compound maintains its inhibitory effects on Rac activation and cell migration over extended periods . In vivo studies have demonstrated that this compound reduces T cell migration to peripheral lymph nodes and decreases lung injury severity over time .
Dosage Effects in Animal Models
The effects of this compound vary with different dosages in animal models. At a dose of 5 mg per animal, this compound significantly reduces T cell migration to peripheral lymph nodes . Higher doses, such as 250 mg/kg, have been shown to reduce MPO activity in lung tissue and decrease cytokine release in serum
Metabolic Pathways
This compound is involved in metabolic pathways that regulate immune responses and inflammation. By inhibiting the GEF activity of DOCK2, DOCK5, and DOCK180, this compound affects the activation of Rac1 and its downstream signaling pathways . This inhibition impacts metabolic flux and metabolite levels, particularly in immune cells such as T and B cells
Transport and Distribution
Within cells and tissues, this compound is transported and distributed through interactions with specific transporters and binding proteins. The compound’s localization and accumulation are influenced by its binding to the DHR-2 domain of DOCK2 . This interaction affects the compound’s distribution within immune cells and its overall efficacy in modulating immune responses .
Subcellular Localization
This compound’s subcellular localization is primarily within the cytoplasm, where it interacts with the DHR-2 domain of DOCK2 . This localization is crucial for its inhibitory effects on Rac1 activation and subsequent cellular processes. The compound’s activity and function are influenced by its targeting signals and post-translational modifications that direct it to specific cellular compartments .
Preparation Methods
Synthetic Routes and Reaction Conditions
The synthesis of CPYPP involves several steps, starting with the preparation of the core pyrazolidinedione structure. The key steps include:
Formation of the pyrazolidinedione core: This is typically achieved through the reaction of phenylhydrazine with ethyl acetoacetate under acidic conditions to form 1-phenyl-3,5-pyrazolidinedione.
Introduction of the chlorophenyl group: The chlorophenyl group is introduced through a condensation reaction with 2-chlorobenzaldehyde in the presence of a base such as sodium hydroxide.
Formation of the propenylidene linkage: The final step involves the formation of the propenylidene linkage through a Knoevenagel condensation reaction with an appropriate aldehyde
Industrial Production Methods
Industrial production of this compound follows similar synthetic routes but is optimized for large-scale production. This involves:
Optimization of reaction conditions: Ensuring high yield and purity by optimizing temperature, solvent, and reaction time.
Purification processes: Utilizing techniques such as recrystallization and chromatography to achieve the desired purity levels
Chemical Reactions Analysis
Types of Reactions
CPYPP undergoes several types of chemical reactions, including:
Oxidation: this compound can be oxidized under specific conditions to form corresponding oxidized products.
Reduction: Reduction reactions can be performed to modify the pyrazolidinedione core or the propenylidene linkage.
Common Reagents and Conditions
Oxidation: Common oxidizing agents include potassium permanganate and hydrogen peroxide.
Reduction: Reducing agents such as sodium borohydride and lithium aluminum hydride are used.
Substitution: Substitution reactions often involve nucleophiles such as amines or thiols under basic conditions
Major Products
The major products formed from these reactions include various derivatives of this compound with modified functional groups, which can be used for further studies and applications .
Scientific Research Applications
CPYPP has a wide range of scientific research applications, including:
Chemistry: Used as a tool compound to study the inhibition of guanine nucleotide exchange factors.
Biology: Employed in research to understand the role of DOCK2-Rac1 interaction in cellular processes such as chemotaxis and cell migration.
Medicine: Investigated for its potential therapeutic applications in diseases where DOCK2-Rac1 interaction plays a critical role, such as autoimmune diseases and cancer.
Industry: Utilized in the development of new inhibitors targeting similar pathways for therapeutic purposes
Comparison with Similar Compounds
Similar Compounds
DOCK180: Another guanine nucleotide exchange factor that interacts with Rac1 but is less inhibited by CPYPP.
DOCK5: Similar to DOCK2, but with different specificity and inhibition profile.
DOCK9: Exhibits less inhibition by this compound compared to DOCK2
Uniqueness of this compound
This compound is unique in its high specificity and potency as a DOCK2-Rac1 interaction inhibitor. It exhibits a dose-dependent inhibition with an IC50 of 22.8 µM, making it a valuable tool for studying the DOCK2-Rac1 pathway .
Properties
IUPAC Name |
(4E)-4-[(E)-3-(2-chlorophenyl)prop-2-enylidene]-1-phenylpyrazolidine-3,5-dione | |
---|---|---|
Source | PubChem | |
URL | https://pubchem.ncbi.nlm.nih.gov | |
Description | Data deposited in or computed by PubChem | |
InChI |
InChI=1S/C18H13ClN2O2/c19-16-12-5-4-7-13(16)8-6-11-15-17(22)20-21(18(15)23)14-9-2-1-3-10-14/h1-12H,(H,20,22)/b8-6+,15-11+ | |
Source | PubChem | |
URL | https://pubchem.ncbi.nlm.nih.gov | |
Description | Data deposited in or computed by PubChem | |
InChI Key |
VVZJFICTTKPNCK-KVDBUQHUSA-N | |
Source | PubChem | |
URL | https://pubchem.ncbi.nlm.nih.gov | |
Description | Data deposited in or computed by PubChem | |
Canonical SMILES |
C1=CC=C(C=C1)N2C(=O)C(=CC=CC3=CC=CC=C3Cl)C(=O)N2 | |
Source | PubChem | |
URL | https://pubchem.ncbi.nlm.nih.gov | |
Description | Data deposited in or computed by PubChem | |
Isomeric SMILES |
C1=CC=C(C=C1)N2C(=O)/C(=C/C=C/C3=CC=CC=C3Cl)/C(=O)N2 | |
Source | PubChem | |
URL | https://pubchem.ncbi.nlm.nih.gov | |
Description | Data deposited in or computed by PubChem | |
Molecular Formula |
C18H13ClN2O2 | |
Source | PubChem | |
URL | https://pubchem.ncbi.nlm.nih.gov | |
Description | Data deposited in or computed by PubChem | |
Molecular Weight |
324.8 g/mol | |
Source | PubChem | |
URL | https://pubchem.ncbi.nlm.nih.gov | |
Description | Data deposited in or computed by PubChem | |
Retrosynthesis Analysis
AI-Powered Synthesis Planning: Our tool employs the Template_relevance Pistachio, Template_relevance Bkms_metabolic, Template_relevance Pistachio_ringbreaker, Template_relevance Reaxys, Template_relevance Reaxys_biocatalysis model, leveraging a vast database of chemical reactions to predict feasible synthetic routes.
One-Step Synthesis Focus: Specifically designed for one-step synthesis, it provides concise and direct routes for your target compounds, streamlining the synthesis process.
Accurate Predictions: Utilizing the extensive PISTACHIO, BKMS_METABOLIC, PISTACHIO_RINGBREAKER, REAXYS, REAXYS_BIOCATALYSIS database, our tool offers high-accuracy predictions, reflecting the latest in chemical research and data.
Strategy Settings
Precursor scoring | Relevance Heuristic |
---|---|
Min. plausibility | 0.01 |
Model | Template_relevance |
Template Set | Pistachio/Bkms_metabolic/Pistachio_ringbreaker/Reaxys/Reaxys_biocatalysis |
Top-N result to add to graph | 6 |
Feasible Synthetic Routes
Q1: What is the primary mechanism of action of Cpypp?
A1: this compound acts as an allosteric inhibitor of specific DOCK proteins, primarily DOCK1 and DOCK5. [, ] These proteins function as atypical guanine nucleotide exchange factors (GEFs) for the Rho GTPases, Rac1 and Cdc42, which are crucial regulators of cytoskeletal dynamics and various cellular processes. [] By inhibiting DOCK1 and DOCK5, this compound disrupts the activation of Rac1 and potentially Cdc42, leading to downstream effects on cell migration, ROS production, and other cellular functions. []
Q2: What are the implications of inhibiting DOCK2 and KCa3.1 with this compound in the context of fibroblast-to-myofibroblast transformation?
A2: Research suggests that both DOCK2 and the potassium channel KCa3.1 play roles in the transformation of fibroblasts into myofibroblasts, a process contributing to fibrosis in various diseases. [] In vitro studies using this compound demonstrated that inhibiting DOCK2 attenuated the upregulation of genes associated with fibroblast transformation induced by transforming growth factor β (TGFβ). [] This suggests that DOCK2, and potentially its downstream signaling pathways targeted by this compound, could be therapeutically relevant in mitigating fibrosis.
Q3: How does this compound impact cancer cells, specifically in the context of the ROBO1 pathway?
A3: Studies indicate that loss of the ROBO1 protein and subsequent elevation of DOCK1 are associated with aggressive prostate cancer, particularly in African American patients. [] this compound, by inhibiting DOCK1, shows promise in preclinical models by decreasing Rac1 activity, inhibiting the transmigration of ROBO1-deficient cancer cells, and reducing metastatic spread. [] These findings suggest that targeting the ROBO1/DOCK1 axis with this compound could hold therapeutic potential for specific cancer subtypes.
Q4: Has this compound been investigated for its role in other cellular processes beyond cell migration and cancer?
A4: Yes, this compound has shown efficacy in modulating neutrophil function. Studies demonstrate that this compound inhibits both fMLF- and PMA-induced Rac activation in neutrophils, leading to impaired chemotaxis and reduced ROS production. [] Additionally, this compound has been implicated in regulating the formation of neutrophil extracellular traps (NETs), which are involved in inflammatory responses. [] These findings suggest a potential role for this compound in modulating neutrophil-mediated inflammation and immune responses.
Disclaimer and Information on In-Vitro Research Products
Please be aware that all articles and product information presented on BenchChem are intended solely for informational purposes. The products available for purchase on BenchChem are specifically designed for in-vitro studies, which are conducted outside of living organisms. In-vitro studies, derived from the Latin term "in glass," involve experiments performed in controlled laboratory settings using cells or tissues. It is important to note that these products are not categorized as medicines or drugs, and they have not received approval from the FDA for the prevention, treatment, or cure of any medical condition, ailment, or disease. We must emphasize that any form of bodily introduction of these products into humans or animals is strictly prohibited by law. It is essential to adhere to these guidelines to ensure compliance with legal and ethical standards in research and experimentation.