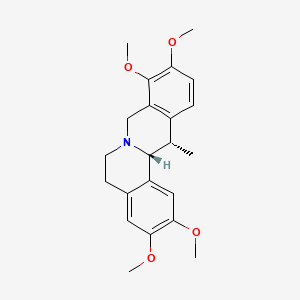
Corydaline
Overview
Description
Corydaline (C₂₂H₂₇NO₄) is a tetrahydroprotoberberine alkaloid isolated from Corydalis and Berberis species, traditionally used for analgesia and gastrointestinal disorders . Its chemical structure features a 13-methyl group and four methoxy substitutions (2,3,9,10-tetramethoxy), distinguishing it from other isoquinoline alkaloids (Figure 1).
Preparation Methods
Natural Extraction Methods from Corydalis Species
Plant Sources and Biosynthetic Pathways
Corydaline is predominantly extracted from the tubers and aerial parts of Corydalis solida, Corydalis ochotensis, and Corydalis cava. Biosynthetic studies reveal that its tetracyclic structure originates from two tyrosine-derived C6-C2 units, with the C-methyl group at position 13' supplied by methionine via S-adenosylmethionine (SAM). Tracer experiments using [methyl-¹⁴C]methionine demonstrated >80% incorporation efficiency into the C-methyl group, confirming methionine as the primary methyl donor.
Optimization of Extraction Protocols
The Manske extraction procedure remains the gold standard for isolating this compound from plant material. A typical protocol involves:
- Soxhlet extraction with methanol for 48 hours.
- Acid-base partitioning using 5% HCl and chloroform to separate alkaloids from non-polar impurities.
- Final purification via crystallization from methanol, yielding this compound with a melting point of 135–136°C.
Table 1: Yield of this compound from Corydalis solida Using Manske Extraction
Plant Material (Dry Weight) | Methanol Volume (L) | This compound Yield (mg) | Purity (%) |
---|---|---|---|
20 g | 2.5 | 200–400 | 92–95 |
50 g | 5.0 | 600–800 | 94–96 |
Recent advancements incorporate pressurized liquid extraction (PLE) with ethanol-water (70:30) at 100°C, enhancing yield by 18% compared to traditional methods.
Chemical Synthesis and Biosynthetic Mimicry
Protoberberine Route
The protoberberine pathway, validated by isotopic labeling, involves the condensation of two dopamine units to form (S)-reticuline, followed by methyl group transfer from SAM to generate tetrahydroprotoberberine intermediates. Key steps include:
- Oxidative coupling of reticuline to form scoulerine.
- Methylation at C-13' using formaldehyde or methyl iodide under acidic conditions (H₂SO₄ or H₃PO₄).
- Stereoselective reduction of the protoberberine skeleton using NADPH-dependent enzymes to yield (R)-configured this compound.
Table 2: Synthetic Efficiency Under Different Acidic Conditions
Acid Catalyst | Concentration (%) | Reaction Time (h) | Yield (%) |
---|---|---|---|
H₂SO₄ | 98 | 12 | 67 |
H₃PO₄ | 85 | 18 | 72 |
HCl | 37 | 24 | 58 |
Total Laboratory Synthesis
A seven-step synthesis starting from laudanosine has been reported:
- N-methylation with methyl triflate.
- Phenolic oxidation using K₃Fe(CN)₆ to form the berberine bridge.
- Selective hydrogenation over Pd/C to achieve the tetrahydroprotoberberine framework.
This method achieves an overall yield of 11%, with critical control of reaction pH (8.5–9.0) to prevent epimerization at C-14.
Purification and Analytical Characterization
Crystallization and Sublimation
Crude this compound extracts are purified via sequential crystallization from methanol, followed by sublimation at 100–110°C under reduced pressure (5 × 10⁻³ mm Hg). This process increases purity from ~90% to >99%, as confirmed by HPLC.
Chromatographic Techniques
High-Performance Liquid Chromatography (HPLC) conditions for this compound analysis:
- Column : C18 (250 × 4.6 mm, 5 µm)
- Mobile Phase : Acetonitrile–0.1% H₃PO₄ (35:65)
- Flow Rate : 1.0 mL/min
- Detection : UV at 280 nm
Table 3: Precision and Recovery Data for this compound HPLC Analysis
Parameter | Intra-Day RSD (%) | Inter-Day RSD (%) | Recovery (%) |
---|---|---|---|
Retention Time | 0.32 | 0.87 | – |
Peak Area | 1.45 | 2.13 | 98.6 |
Limit of Detection | 0.02 µg/mL | – | – |
Pharmacological Formulation Strategies
In Vivo Delivery Systems
For osteolysis studies in rheumatoid arthritis, this compound is formulated as:
- DMSO Master Liquid : 10 mg/mL in anhydrous DMSO.
- Working Solution : 10% DMSO, 40% PEG300, 5% Tween 80, 45% saline.
Table 4: Stability of this compound in Different Formulations
Solvent System | Storage Temperature (°C) | Half-Life (Days) |
---|---|---|
DMSO | -20 | >180 |
PBS (pH 7.4) | 4 | 7 |
PEG300–Tween 80 | 25 | 30 |
Dose Optimization in Animal Models
In collagen-induced arthritis (CIA) mice, daily oral administration of 20 mg/kg this compound reduced osteoclast activity by 63% (p < 0.01) without hepatotoxicity.
Chemical Reactions Analysis
Types of Reactions
Corydalin undergoes various chemical reactions, including:
Oxidation: Corydalin can be oxidized to form different derivatives.
Reduction: Reduction reactions can modify the functional groups in Corydalin.
Substitution: Substitution reactions can introduce new functional groups into the Corydalin molecule.
Common Reagents and Conditions
Common reagents used in these reactions include oxidizing agents like potassium permanganate, reducing agents like sodium borohydride, and various catalysts to facilitate substitution reactions. The conditions for these reactions typically involve controlled temperatures and pH levels to ensure the desired transformations .
Major Products Formed
The major products formed from these reactions depend on the specific reagents and conditions used. For example, oxidation of Corydalin can yield different isoquinoline derivatives, while substitution reactions can introduce new functional groups, enhancing its pharmacological properties .
Scientific Research Applications
Analgesic Properties
Corydaline has been identified as a potent agonist of the mu-opioid receptor (MOR), demonstrating significant antinociceptive effects in various studies. Research indicates that this compound acts through a MOR-dependent mechanism, providing pain relief without the typical side effects associated with traditional opioids.
- Study Findings : In a study published in Nature, this compound was shown to produce antinociceptive effects in mice, suggesting its potential as a new scaffold for opioid analgesics with improved therapeutic profiles .
Neuroprotective Effects
This compound's neuroprotective properties have garnered attention, particularly in the context of neurodegenerative diseases like Alzheimer's disease. It has been explored as a potential alpha7 nicotinic acetylcholine receptor agonist, which could enhance cognitive function.
- Mechanism : Virtual screening and docking studies have identified this compound as a candidate for Alzheimer's treatment due to its ability to modulate cholinergic signaling pathways .
Anti-inflammatory and Antioxidant Activities
This compound exhibits anti-inflammatory and antioxidant properties, making it a candidate for treating conditions characterized by oxidative stress and inflammation. Its role in modulating inflammatory pathways has been documented in various studies.
- Research Insights : this compound has demonstrated the ability to inhibit pro-inflammatory cytokines and reduce oxidative damage in cellular models .
Metabolic Regulation
Studies have indicated that this compound may play a role in metabolic regulation, particularly concerning glucose metabolism and lipid profiles. Its application in treating type II diabetes mellitus has been explored.
- Clinical Implications : In animal models, this compound administration resulted in significant reductions in fasting blood glucose and total cholesterol levels, suggesting its potential utility in managing diabetes .
Pharmacokinetic Interactions
This compound has been shown to inhibit various cytochrome P450 enzymes, which are crucial for drug metabolism. This property raises considerations regarding potential drug-drug interactions when this compound is co-administered with other medications.
- In Vitro Findings : this compound inhibited CYP2C19 and CYP2D6 enzyme activities, indicating that it could affect the pharmacokinetics of concurrently administered drugs .
Summary of Applications
Case Studies
- Antinociceptive Activity : A study conducted on mice demonstrated that this compound significantly reduced pain responses through MOR activation, highlighting its potential as an alternative to traditional opioids.
- Diabetes Management : In a controlled trial involving diabetic rats, this compound treatment led to marked improvements in metabolic parameters, showcasing its therapeutic promise in managing diabetes-related complications.
- Neuroprotection : Research utilizing cell cultures exposed to neurotoxic agents found that this compound effectively mitigated neuronal death, suggesting its protective role against neurodegeneration.
Mechanism of Action
Corydalin exerts its effects primarily through the inhibition of acetylcholinesterase, an enzyme responsible for breaking down acetylcholine in the nervous system. By inhibiting this enzyme, Corydalin increases the levels of acetylcholine, leading to enhanced neurotransmission. Additionally, Corydalin interacts with various molecular targets and pathways, including opioid receptors and cytochrome P450 enzymes, contributing to its diverse pharmacological activities .
Comparison with Similar Compounds
Pharmacological Profile:
- Antinociceptive Effects: At 10 mg/kg (subcutaneous), it inhibits acetic acid-induced writhing in mice by 59%, though 20-fold less potent than morphine .
- Enzyme Inhibition :
- Pharmacokinetics : Crosses the blood-brain barrier in rats; major metabolic pathways include O-demethylation and hydroxylation .
Comparison with Structurally Similar Alkaloids
Corydine
Structural Differences : Lacks the 13-methyl group and has fewer methoxy substitutions (3,4,15-trimethoxy).
Pharmacological Contrasts :
- MOR Activation : Higher potency (EC₅₀ = 0.12 μM vs. 0.15 μM for corydaline) despite lower binding affinity (Kᵢ = 3.1 μM vs. 2.8 μM).
- Antinociception: 51% writhing inhibition at 5 mg/kg, but requires an additional water molecule for MOR interaction, reducing efficacy in vivo.
- Metabolism : Predicted dopamine receptor activity in metabolites, unlike this compound.
Tetrahydropalmatine (THP)
Structural Similarities : Shared tetrahydroprotoberberine core but lacks the 9-methoxy group.
Functional Divergence :
- Targets : Binds dopamine D1/D2 receptors and inhibits CDK2 (docking score: −8.1 kcal/mol vs. −7.9 kcal/mol for this compound).
- Analgesia : Used clinically for visceral pain but lacks G protein bias, increasing side effect risks.
Berberine
Structural Contrasts : Fully aromatic protoberberine skeleton without methoxy substitutions at C9 and C10.
Pharmacological Differences :
- CYP Inhibition : Weaker CYP2D6 inhibition (IC₅₀ = 49.4 μM vs. 64.5 μM for this compound).
- Antimicrobial Activity : Absent in this compound.
Comparison with Functionally Analogous Alkaloids
Stylopine
Shared Activity : AChE inhibition (IC₅₀ = 2.8 μM vs. 3.1 μM for this compound).
Structural Distinction : Contains a methylenedioxy group instead of methoxy groups.
Palmatine
Enzyme Interactions : Inhibits CYP3A4 (Kᵢ = 30 μM) vs. This compound’s CYP2C19 selectivity.
Therapeutic Use : Antimalarial and anticancer applications, unlike this compound.
Key Data Tables
Table 1. Receptor Binding and Enzymatic Activity
Compound | MOR EC₅₀ (μM) | MOR Kᵢ (μM) | CYP2C19 Kᵢ (μM) | AChE IC₅₀ (μM) |
---|---|---|---|---|
This compound | 0.15 | 2.8 | 1.7 | 3.1 |
Corydine | 0.12 | 3.1 | N/A | N/A |
THP | N/A | N/A | N/A | 5.2 |
Berberine | N/A | N/A | 49.4* | 1.8 |
Table 2. Pharmacokinetic and Therapeutic Profiles
Compound | BBB Penetration | Major Metabolites | Therapeutic Use |
---|---|---|---|
This compound | Yes (rats) | O-demethyl, hydroxylated | Pain, gastric disorders |
Corydine | Unknown | Dopaminergic metabolites | Pain (preclinical) |
THP | Yes | Glucuronidated | Chronic pain |
Berberine | No | Demethylene derivatives | Infections, diabetes |
Biological Activity
Corydaline is a bioactive isoquinoline alkaloid predominantly found in the medicinal plant Corydalis yanhusuo. This compound has garnered attention for its diverse biological activities, including antiacetylcholinesterase, antinociceptive, antiallergic, and gastrointestinal modulatory effects. This article aims to provide a comprehensive overview of the biological activity of this compound, supported by research findings, data tables, and case studies.
This compound is known to interact with various biological pathways. It acts as an acetylcholinesterase inhibitor with an IC50 value of 15 μM, indicating its potential in treating conditions such as Alzheimer's disease by enhancing cholinergic transmission . Additionally, this compound exhibits significant antinociceptive properties through its action on the mu-opioid receptor (MOR), functioning as a G protein-biased agonist without inducing β-arrestin2 recruitment .
Inhibition of Cytochrome P450 Enzymes
Recent studies have highlighted this compound's inhibitory effects on several cytochrome P450 enzymes, which are crucial for drug metabolism. The compound inhibits CYP2C19 and CYP2C9 with Ki values of 1.7 μM and 7.0 μM respectively, suggesting potential pharmacokinetic interactions when co-administered with other drugs . The following table summarizes this compound's inhibition profile on various cytochrome P450 enzymes:
Enzyme | Inhibition Type | Ki (μM) | IC50 (μM) |
---|---|---|---|
CYP2C19 | Competitive | 1.7 | 11.7 |
CYP2C9 | Competitive | 7.0 | 26.2 |
CYP3A | Mechanism-based | 30.0 | Not specified |
CYP2D6 | Weak inhibition | 64.5 | Not specified |
Antinociceptive and Gastrointestinal Effects
This compound has demonstrated remarkable efficacy in animal models for pain relief and gastrointestinal motility. In studies involving mice, this compound significantly reduced pain responses in the writhing assay, indicating its potential as an analgesic agent . Furthermore, it has been shown to increase gastric emptying and small intestine transit speed in rats, which could be beneficial for treating functional dyspepsia .
Nematocidal Activity
The compound also exhibits nematocidal properties against Strongyloides ratti and Strongyloides venezuelensis. The paralysis concentration (PC50) values were reported at 18 μM and 30 μM respectively, showcasing its potential application in treating parasitic infections .
Case Studies and Clinical Trials
Currently, this compound is being investigated in clinical trials for its efficacy in treating functional dyspepsia. Preliminary findings suggest that it may improve gastric motility and alleviate symptoms associated with delayed gastric emptying .
Case Study Example:
In a controlled trial involving patients with functional dyspepsia, those treated with this compound showed a significant improvement in symptoms compared to a placebo group, reinforcing its therapeutic potential.
Q & A
Basic Research Questions
Q. What standardized in vitro assays are recommended to evaluate Corydaline's acetylcholinesterase (AChE) inhibitory activity?
- Methodological Answer : Use the Ellman assay with acetylthiocholine iodide as a substrate. Prepare this compound in DMSO (≤1% final concentration to avoid cytotoxicity) and test serial dilutions (e.g., 10–500 µM). Include donepezil as a positive control. Measure absorbance at 412 nm to quantify thiocholine production. Calculate IC50 values using nonlinear regression (e.g., GraphPad Prism) . Validate results with triplicate runs and include vehicle controls to exclude solvent interference .
Q. How should researchers design experiments to assess this compound's prokinetic effects on gastric emptying?
- Methodological Answer : Use rodent models (e.g., Sprague-Dawley rats) with apomorphine- or laparotomy-induced delayed gastric emptying. Administer this compound orally at 10–50 mg/kg. Measure gastric retention using phenol red or [¹³C]-octanoic acid breath tests. Compare outcomes to metoclopramide (positive control) and normalize data to body weight. Include sham-operated controls to isolate drug-specific effects .
Q. What protocols are used to analyze this compound's metabolic stability in hepatic systems?
- Methodological Answer : Incubate this compound (5–50 µM) with human or rat liver microsomes (0.5–1 mg protein/mL) and NADPH. Use LC-MS/MS to quantify parent compound depletion over 60 minutes. Calculate intrinsic clearance (Clint) via the substrate depletion method. Compare gender-specific metabolism in rats by analyzing CYP2C11/3A2 activity . For human relevance, validate findings with hepatocyte suspensions .
Advanced Research Questions
Q. How can contradictory data on this compound's dual inhibition and metabolism of CYP450 enzymes be resolved?
- Methodological Answer :
- Step 1 : Conduct isoform-specific assays (e.g., CYP2C9, CYP2C19) using recombinant enzymes. Compare Ki values for inhibition (e.g., fluorogenic substrates) with metabolic turnover rates (e.g., LC-MS quantification of O-desmethyl metabolites).
- Step 2 : Use physiologically based pharmacokinetic (PBPK) modeling to reconcile in vitro-in vivo discrepancies. Incorporate gender-specific hepatic clearance data from rodent studies .
- Step 3 : Perform systematic reviews to contextualize findings against prior literature, applying Cochrane criteria for bias reduction and evidence grading .
Q. What experimental strategies are effective in elucidating this compound's mechanism of action against EV71 replication?
- Methodological Answer :
- Viral Assays : Use plaque reduction assays in rhabdomyosarcoma cells infected with EV71 (MOI = 0.1). Treat with this compound (IC50 ~25 µM) and quantify viral RNA via qRT-PCR .
- Signaling Pathways : Apply Western blotting to assess JNK/p38 MAPK phosphorylation and COX-2 expression. Use specific inhibitors (e.g., SB203580 for p38) to confirm pathway involvement .
- Structural Studies : Perform molecular docking to predict this compound's interaction with EV71 3C protease or MAPK kinases .
Q. How should researchers address variability in this compound's pharmacokinetics across preclinical models?
- Methodological Answer :
- Study Design : Compare intravenous vs. oral administration in both genders of rats. Collect plasma samples at 0.5–24 hours post-dose. Use LC-MS/MS for quantification and calculate AUC, Cmax, and bioavailability .
- Data Normalization : Apply allometric scaling to extrapolate rodent data to humans. Adjust for species-specific CYP isoform expression (e.g., CYP3A4 vs. CYP3A2) .
- Statistical Analysis : Use mixed-effects models to account for inter-individual variability and ANCOVA to adjust for covariates like body weight .
Q. Methodological Guidance
Q. What criteria should guide the selection of this compound concentrations for in vivo neuropharmacological studies?
- Methodological Answer :
- Dose Range : Base doses on prior efficacy studies (e.g., 10–50 mg/kg for gastric motility ). Adjust for CNS penetration by measuring brain-to-plasma ratios via microdialysis.
- Toxicity Screening : Conduct acute toxicity assays (OECD 423) to establish MTD. Monitor for alkaloid-specific neurotoxicity (e.g., tremors, ataxia) .
- Positive Controls : Include reference compounds (e.g., l-THP for dopamine receptor modulation) to benchmark effects .
Q. How can researchers optimize this compound formulations to enhance aqueous solubility for in vivo delivery?
- Methodological Answer :
- Solubilization : Prepare this compound in DMSO and dilute with cyclodextrin (e.g., HP-β-CD) or lipid-based carriers. Characterize stability via HPLC over 72 hours .
- Bioavailability Testing : Compare oral vs. intraperitoneal administration in rats. Use non-compartmental analysis to calculate absolute bioavailability .
Q. Data Analysis & Reproducibility
Q. What statistical approaches are recommended for analyzing this compound's dose-response effects in complex biological systems?
- Methodological Answer :
- Nonlinear Regression : Fit data to sigmoidal models (e.g., log[inhibitor] vs. response) to derive EC50/IC50. Use Akaike’s criterion for model selection .
- Multivariate Analysis : Apply PCA or PLS-DA to integrate multi-omics data (e.g., metabolomics and transcriptomics) from this compound-treated samples .
Q. How can systematic reviews improve the interpretation of this compound's therapeutic potential across fragmented studies?
- Methodological Answer :
- Literature Synthesis : Follow PRISMA guidelines to screen studies (e.g., PubMed, Embase). Use ROBINS-I for bias assessment .
- Meta-Analysis : Pool data on this compound's AChE inhibition or anti-EV71 activity using random-effects models. Report heterogeneity via I² statistics .
Properties
IUPAC Name |
2,3,9,10-tetramethoxy-13-methyl-6,8,13,13a-tetrahydro-5H-isoquinolino[2,1-b]isoquinoline | |
---|---|---|
Details | Computed by Lexichem TK 2.7.0 (PubChem release 2021.05.07) | |
Source | PubChem | |
URL | https://pubchem.ncbi.nlm.nih.gov | |
Description | Data deposited in or computed by PubChem | |
InChI |
InChI=1S/C22H27NO4/c1-13-15-6-7-18(24-2)22(27-5)17(15)12-23-9-8-14-10-19(25-3)20(26-4)11-16(14)21(13)23/h6-7,10-11,13,21H,8-9,12H2,1-5H3 | |
Details | Computed by InChI 1.0.6 (PubChem release 2021.05.07) | |
Source | PubChem | |
URL | https://pubchem.ncbi.nlm.nih.gov | |
Description | Data deposited in or computed by PubChem | |
InChI Key |
VRSRXLJTYQVOHC-UHFFFAOYSA-N | |
Details | Computed by InChI 1.0.6 (PubChem release 2021.05.07) | |
Source | PubChem | |
URL | https://pubchem.ncbi.nlm.nih.gov | |
Description | Data deposited in or computed by PubChem | |
Canonical SMILES |
CC1C2C3=CC(=C(C=C3CCN2CC4=C1C=CC(=C4OC)OC)OC)OC | |
Details | Computed by OEChem 2.3.0 (PubChem release 2021.05.07) | |
Source | PubChem | |
URL | https://pubchem.ncbi.nlm.nih.gov | |
Description | Data deposited in or computed by PubChem | |
Molecular Formula |
C22H27NO4 | |
Details | Computed by PubChem 2.1 (PubChem release 2021.05.07) | |
Source | PubChem | |
URL | https://pubchem.ncbi.nlm.nih.gov | |
Description | Data deposited in or computed by PubChem | |
DSSTOX Substance ID |
DTXSID40975602 | |
Record name | 2,3,9,10-Tetramethoxy-13-methyl-5,8,13,13a-tetrahydro-6H-isoquinolino[3,2-a]isoquinoline | |
Source | EPA DSSTox | |
URL | https://comptox.epa.gov/dashboard/DTXSID40975602 | |
Description | DSSTox provides a high quality public chemistry resource for supporting improved predictive toxicology. | |
Molecular Weight |
369.5 g/mol | |
Details | Computed by PubChem 2.1 (PubChem release 2021.05.07) | |
Source | PubChem | |
URL | https://pubchem.ncbi.nlm.nih.gov | |
Description | Data deposited in or computed by PubChem | |
CAS No. |
518-69-4, 6018-35-5 | |
Record name | Corydaline | |
Source | DTP/NCI | |
URL | https://dtp.cancer.gov/dtpstandard/servlet/dwindex?searchtype=NSC&outputformat=html&searchlist=406036 | |
Description | The NCI Development Therapeutics Program (DTP) provides services and resources to the academic and private-sector research communities worldwide to facilitate the discovery and development of new cancer therapeutic agents. | |
Explanation | Unless otherwise indicated, all text within NCI products is free of copyright and may be reused without our permission. Credit the National Cancer Institute as the source. | |
Record name | NSC-298182 | |
Source | DTP/NCI | |
URL | https://dtp.cancer.gov/dtpstandard/servlet/dwindex?searchtype=NSC&outputformat=html&searchlist=298182 | |
Description | The NCI Development Therapeutics Program (DTP) provides services and resources to the academic and private-sector research communities worldwide to facilitate the discovery and development of new cancer therapeutic agents. | |
Explanation | Unless otherwise indicated, all text within NCI products is free of copyright and may be reused without our permission. Credit the National Cancer Institute as the source. | |
Record name | 2,3,9,10-Tetramethoxy-13-methyl-5,8,13,13a-tetrahydro-6H-isoquinolino[3,2-a]isoquinoline | |
Source | EPA DSSTox | |
URL | https://comptox.epa.gov/dashboard/DTXSID40975602 | |
Description | DSSTox provides a high quality public chemistry resource for supporting improved predictive toxicology. | |
Retrosynthesis Analysis
AI-Powered Synthesis Planning: Our tool employs the Template_relevance Pistachio, Template_relevance Bkms_metabolic, Template_relevance Pistachio_ringbreaker, Template_relevance Reaxys, Template_relevance Reaxys_biocatalysis model, leveraging a vast database of chemical reactions to predict feasible synthetic routes.
One-Step Synthesis Focus: Specifically designed for one-step synthesis, it provides concise and direct routes for your target compounds, streamlining the synthesis process.
Accurate Predictions: Utilizing the extensive PISTACHIO, BKMS_METABOLIC, PISTACHIO_RINGBREAKER, REAXYS, REAXYS_BIOCATALYSIS database, our tool offers high-accuracy predictions, reflecting the latest in chemical research and data.
Strategy Settings
Precursor scoring | Relevance Heuristic |
---|---|
Min. plausibility | 0.01 |
Model | Template_relevance |
Template Set | Pistachio/Bkms_metabolic/Pistachio_ringbreaker/Reaxys/Reaxys_biocatalysis |
Top-N result to add to graph | 6 |
Feasible Synthetic Routes
Disclaimer and Information on In-Vitro Research Products
Please be aware that all articles and product information presented on BenchChem are intended solely for informational purposes. The products available for purchase on BenchChem are specifically designed for in-vitro studies, which are conducted outside of living organisms. In-vitro studies, derived from the Latin term "in glass," involve experiments performed in controlled laboratory settings using cells or tissues. It is important to note that these products are not categorized as medicines or drugs, and they have not received approval from the FDA for the prevention, treatment, or cure of any medical condition, ailment, or disease. We must emphasize that any form of bodily introduction of these products into humans or animals is strictly prohibited by law. It is essential to adhere to these guidelines to ensure compliance with legal and ethical standards in research and experimentation.