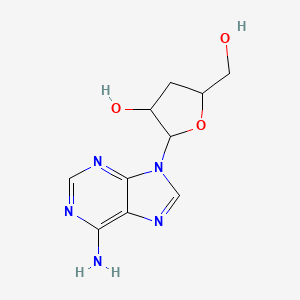
Cordycepin
Overview
Description
. It has garnered significant attention due to its diverse biological activities and potential therapeutic applications.
Mechanism of Action
Target of Action
Cordycepin, a nucleoside analog derived from the fungus Cordyceps, has been found to interact with several key targets in the cell. These targets include catalase (CAT) , CREB binding protein (CREBBP) , epidermal growth factor (EGF) , and E1A binding protein P300 (EP300) . These proteins play crucial roles in various cellular processes, including signal transduction, gene expression, and cell proliferation .
Mode of Action
This compound exerts its effects by interacting with these targets and modulating their activity. For instance, it has been found to inhibit the PI3K/mTOR/AKT and ERK signaling pathways, while activating AMPK . This leads to a reduction in cell proliferation and migration, as well as a decrease in inflammation . Furthermore, this compound’s structural similarity to adenosine allows it to interfere with RNA synthesis, leading to premature termination of transcription .
Biochemical Pathways
This compound affects several biochemical pathways. It consistently reduces PI3K/mTOR/AKT and ERK signaling, leading to decreased cell survival and proliferation . It also activates AMPK, a key regulator of cellular energy homeostasis . The effects of this compound on other kinases such as p38 and jun are variable, suggesting these are cell-specific responses .
Pharmacokinetics
The absorption, distribution, metabolism, and excretion (ADME) characteristics of this compound directly influence its bioavailability and therapeutic levels . Understanding these pharmacokinetic properties is crucial for optimizing its effectiveness in clinical settings .
Result of Action
This compound has a wide range of molecular and cellular effects. It can induce apoptosis, resist cell cycle progression, and cause DNA damage in cancer cells, thereby controlling cancer cell growth . It can also induce autophagy and modulate the immune system . Furthermore, this compound inhibits tumor metastasis .
Action Environment
Environmental factors can influence the action, efficacy, and stability of this compound. For instance, the fungus Cordyceps, from which this compound is derived, has been found to thrive in specific environmental conditions, and these conditions could potentially affect the production and potency of this compound
Biochemical Analysis
Biochemical Properties
Cordycepin is similar to adenosine, and some enzymes cannot discriminate between the two . It can participate in certain biochemical reactions, such as triggering the premature termination of mRNA synthesis . By acting as an adenosine analog, this compound was found to be the most potent molecular circadian clock resetter out of several screened compounds .
Cellular Effects
This compound has displayed cytotoxicity against some leukemic cell lines in vitro . It has also shown effects in other types of cancers, such as lung, renal, colon, and breast cancer . This compound has been shown to reduce viable A549 lung cancer cell populations by 50% . It has also been found to produce rapid, robust antidepressant effects in animal models of depression .
Molecular Mechanism
This compound is an activator of adenosine receptors . It enhances human immunity, promotes anti-inflammatory processes, inhibits RNA virus reproduction, and protects against brain, lung, liver, heart, and kidney damage . The main pathway of this compound biosynthesis involves the phosphorylation of adenosine to 3′-AMP by nucleotide kinase (Cns3), then dephosphorylation to 2′-C-3′-dA by phosphohydrolase (Cns2), and this compound is finally produced by oxidoreductase (Cns1) .
Temporal Effects in Laboratory Settings
This compound has been found to produce rapid and robust antidepressant effects, which were significantly faster and stronger than imipramine, after 45 minutes in tail suspension and forced swim tests . This antidepressant effect remained after 5 days of treatment with this compound .
Dosage Effects in Animal Models
In animal studies, this compound has shown many potential therapeutic effects, including the reduction of tumor growth, repression of pain and inflammation, protecting brain function, improvement of respiratory and cardiac conditions, and amelioration of metabolic disorders .
Metabolic Pathways
The main pathway of this compound biosynthesis is clear. Adenosine is phosphorylated to 3′-AMP by nucleotide kinase (Cns3), then dephosphorylated to 2′-C-3′-dA by phosphohydrolase (Cns2), and this compound is finally produced by oxidoreductase (Cns1) .
Transport and Distribution
This compound, as an activator of adenosine receptors, can enhance human immunity, promote anti-inflammatory processes, inhibit RNA virus reproduction, protect against brain, lung, liver, heart, and kidney damage, and ameliorate lung-fibrosis in clinical and animal models .
Preparation Methods
Synthetic Routes and Reaction Conditions
The synthesis of 3’-Deoxyadenosine typically involves the selective deoxygenation of adenosine at the 3’ position. One common method includes the use of reagents such as triphenylphosphine and diethyl azodicarboxylate (DEAD) in the presence of a suitable solvent like tetrahydrofuran (THF). The reaction is carried out under an inert atmosphere, usually nitrogen, at low temperatures to ensure high yield and purity .
Industrial Production Methods
Industrial production of 3’-Deoxyadenosine often employs biotechnological approaches, utilizing microbial fermentation processes. The fungus Cordyceps militaris is cultured under controlled conditions to produce the compound in large quantities. This method is preferred due to its cost-effectiveness and sustainability .
Chemical Reactions Analysis
Types of Reactions
3’-Deoxyadenosine undergoes various chemical reactions, including:
Oxidation: It can be oxidized to form 3’-Deoxyinosine.
Reduction: Reduction reactions can modify its functional groups, enhancing its biological activity.
Substitution: Nucleophilic substitution reactions can introduce different substituents at the 3’ position, altering its properties.
Common Reagents and Conditions
Oxidation: Reagents like potassium permanganate (KMnO4) or hydrogen peroxide (H2O2) are commonly used.
Reduction: Sodium borohydride (NaBH4) or lithium aluminum hydride (LiAlH4) are typical reducing agents.
Substitution: Nucleophiles such as halides or amines are used under basic conditions to achieve substitution.
Major Products
Oxidation: 3’-Deoxyinosine
Reduction: Various reduced derivatives with enhanced biological activity
Substitution: Substituted analogs with modified pharmacological properties
Scientific Research Applications
3’-Deoxyadenosine has a wide range of scientific research applications:
Chemistry: Used as a building block in the synthesis of nucleoside analogs.
Biology: Studied for its role in cellular processes and as a tool to investigate nucleic acid metabolism.
Medicine: Explored for its potential as an anticancer, antiviral, and anti-inflammatory agent.
Industry: Utilized in the production of pharmaceuticals and as a bioactive compound in health supplements.
Comparison with Similar Compounds
Similar Compounds
Adenosine: A naturally occurring nucleoside with similar structure but different biological activity.
2’-Deoxyadenosine: Another nucleoside analog with distinct pharmacological properties.
Cordycepin analogs: Various synthetic derivatives of 3’-Deoxyadenosine with modified activity.
Uniqueness
3’-Deoxyadenosine is unique due to its specific deoxygenation at the 3’ position, which imparts distinct biological activities compared to other nucleoside analogs. Its ability to interfere with RNA synthesis and its broad spectrum of biological effects make it a valuable compound in scientific research and therapeutic applications .
Properties
IUPAC Name |
2-(6-aminopurin-9-yl)-5-(hydroxymethyl)oxolan-3-ol | |
---|---|---|
Details | Computed by Lexichem TK 2.7.0 (PubChem release 2021.05.07) | |
Source | PubChem | |
URL | https://pubchem.ncbi.nlm.nih.gov | |
Description | Data deposited in or computed by PubChem | |
InChI |
InChI=1S/C10H13N5O3/c11-8-7-9(13-3-12-8)15(4-14-7)10-6(17)1-5(2-16)18-10/h3-6,10,16-17H,1-2H2,(H2,11,12,13) | |
Details | Computed by InChI 1.0.6 (PubChem release 2021.05.07) | |
Source | PubChem | |
URL | https://pubchem.ncbi.nlm.nih.gov | |
Description | Data deposited in or computed by PubChem | |
InChI Key |
OFEZSBMBBKLLBJ-UHFFFAOYSA-N | |
Details | Computed by InChI 1.0.6 (PubChem release 2021.05.07) | |
Source | PubChem | |
URL | https://pubchem.ncbi.nlm.nih.gov | |
Description | Data deposited in or computed by PubChem | |
Canonical SMILES |
C1C(OC(C1O)N2C=NC3=C(N=CN=C32)N)CO | |
Details | Computed by OEChem 2.3.0 (PubChem release 2021.05.07) | |
Source | PubChem | |
URL | https://pubchem.ncbi.nlm.nih.gov | |
Description | Data deposited in or computed by PubChem | |
Molecular Formula |
C10H13N5O3 | |
Details | Computed by PubChem 2.1 (PubChem release 2021.05.07) | |
Source | PubChem | |
URL | https://pubchem.ncbi.nlm.nih.gov | |
Description | Data deposited in or computed by PubChem | |
DSSTOX Substance ID |
DTXSID00859090 | |
Record name | 9-(3-Deoxypentofuranosyl)-9H-purin-6-amine | |
Source | EPA DSSTox | |
URL | https://comptox.epa.gov/dashboard/DTXSID00859090 | |
Description | DSSTox provides a high quality public chemistry resource for supporting improved predictive toxicology. | |
Molecular Weight |
251.24 g/mol | |
Details | Computed by PubChem 2.1 (PubChem release 2021.05.07) | |
Source | PubChem | |
URL | https://pubchem.ncbi.nlm.nih.gov | |
Description | Data deposited in or computed by PubChem | |
CAS No. |
73-03-0 | |
Record name | Cordycepin | |
Source | DTP/NCI | |
URL | https://dtp.cancer.gov/dtpstandard/servlet/dwindex?searchtype=NSC&outputformat=html&searchlist=401022 | |
Description | The NCI Development Therapeutics Program (DTP) provides services and resources to the academic and private-sector research communities worldwide to facilitate the discovery and development of new cancer therapeutic agents. | |
Explanation | Unless otherwise indicated, all text within NCI products is free of copyright and may be reused without our permission. Credit the National Cancer Institute as the source. | |
Record name | Cordycepin | |
Source | DTP/NCI | |
URL | https://dtp.cancer.gov/dtpstandard/servlet/dwindex?searchtype=NSC&outputformat=html&searchlist=63984 | |
Description | The NCI Development Therapeutics Program (DTP) provides services and resources to the academic and private-sector research communities worldwide to facilitate the discovery and development of new cancer therapeutic agents. | |
Explanation | Unless otherwise indicated, all text within NCI products is free of copyright and may be reused without our permission. Credit the National Cancer Institute as the source. | |
Retrosynthesis Analysis
AI-Powered Synthesis Planning: Our tool employs the Template_relevance Pistachio, Template_relevance Bkms_metabolic, Template_relevance Pistachio_ringbreaker, Template_relevance Reaxys, Template_relevance Reaxys_biocatalysis model, leveraging a vast database of chemical reactions to predict feasible synthetic routes.
One-Step Synthesis Focus: Specifically designed for one-step synthesis, it provides concise and direct routes for your target compounds, streamlining the synthesis process.
Accurate Predictions: Utilizing the extensive PISTACHIO, BKMS_METABOLIC, PISTACHIO_RINGBREAKER, REAXYS, REAXYS_BIOCATALYSIS database, our tool offers high-accuracy predictions, reflecting the latest in chemical research and data.
Strategy Settings
Precursor scoring | Relevance Heuristic |
---|---|
Min. plausibility | 0.01 |
Model | Template_relevance |
Template Set | Pistachio/Bkms_metabolic/Pistachio_ringbreaker/Reaxys/Reaxys_biocatalysis |
Top-N result to add to graph | 6 |
Feasible Synthetic Routes
Disclaimer and Information on In-Vitro Research Products
Please be aware that all articles and product information presented on BenchChem are intended solely for informational purposes. The products available for purchase on BenchChem are specifically designed for in-vitro studies, which are conducted outside of living organisms. In-vitro studies, derived from the Latin term "in glass," involve experiments performed in controlled laboratory settings using cells or tissues. It is important to note that these products are not categorized as medicines or drugs, and they have not received approval from the FDA for the prevention, treatment, or cure of any medical condition, ailment, or disease. We must emphasize that any form of bodily introduction of these products into humans or animals is strictly prohibited by law. It is essential to adhere to these guidelines to ensure compliance with legal and ethical standards in research and experimentation.