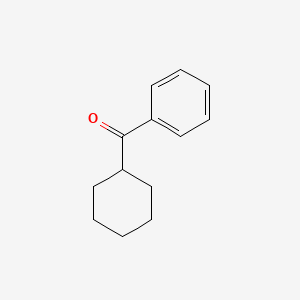
Cyclohexyl phenyl ketone
Overview
Description
Cyclohexyl phenyl ketone (IUPAC: Cyclohexyl(phenyl)methanone, CAS 712-50-5) is an aromatic ketone with the molecular formula C₁₃H₁₆O. Structurally, it consists of a cyclohexyl group attached to a benzophenone scaffold. This compound is synthesized via Friedel-Crafts acylation or catalytic methods using MnO, achieving yields up to 56.4% under optimized conditions (345°C, 4 hours) . Key applications include:
- Photochemistry: Precursor to α-hydroxythis compound (Irgacure 184), a high-efficiency photoinitiator used in coatings and electronics .
- Polymer Science: Incorporation into poly(aryl ether ketone) resins (PAEK) reduces dielectric constants (2.95–3.26 @10 GHz), enhancing suitability for high-frequency communication devices .
- Organic Synthesis: Intermediate in catalytic enantioselective reactions, such as cyanosilylation and hydrosilylation, with enantiomeric excess (ee) up to 83% .
Preparation Methods
Diels-Alder-Based Continuous Synthesis
Reaction Mechanism and Stepwise Procedure
The most efficient modern method, as detailed in US Patent 6,881,865, involves a four-step continuous process conducted in a single reactor without intermediate purification. The sequence begins with a [2+4] Diels-Alder reaction between 1,3-butadiene and acrylic acid, forming 3-cyclohexene-1-carboxylic acid. This step proceeds at 120–150°C in benzene or non-aromatic solvents like hexane, achieving >99% conversion of acrylic acid.
Subsequent hydrogenation of the Diels-Alder adduct over a palladium catalyst saturates the cyclohexene ring, yielding cyclohexanecarboxylic acid. The reaction occurs at 50–100°C under 10–30 bar hydrogen pressure, maintaining near-quantitative conversion.
In the chlorination step , thionyl chloride (1.5–2.0 molar equivalents) converts cyclohexanecarboxylic acid to cyclohexanecarbonyl chloride at 25–50°C. Gas chromatography (GC) analyses confirm >99% selectivity, with phosphorous trichloride serving as an alternative chlorinating agent.
Finally, a Friedel-Crafts acylation introduces the phenyl group via reaction with benzene and anhydrous AlCl₃ (1.5:1 molar ratio to acyl chloride). This step achieves >99% selectivity for cyclohexyl phenyl ketone at reflux temperatures, eliminating the need for solvent removal.
Optimization Parameters
Key variables influencing yield include:
Chlorinating Agent Stoichiometry
As shown in Table 1, increasing the thionyl chloride-to-acid ratio from 1.0:1.0 to 1.5:1.0 elevates conversion from 89% to >99%, while selectivity remains consistently high.
Cyclohexanecarboxylic Acid : Thionyl Chloride (Molar Ratio) | Conversion Rate (%) | Selectivity (%) |
---|---|---|
1.0 : 1.0 | 89 | >99 |
1.0 : 1.5 | >99 | >99 |
Catalyst Loading in Friedel-Crafts Step
AlCl₃ must exceed stoichiometric amounts to ensure complete acylation. At a 1.5:1 AlCl₃-to-acyl chloride ratio, conversion reaches >99%, compared to 74% at 1.0:1 (Table 2).
Cyclohexanecarbonyl Chloride : AlCl₃ (Molar Ratio) | Conversion Rate (%) | Selectivity (%) |
---|---|---|
1.0 : 1.0 | 74 | >99 |
1.0 : 1.5 | >99 | >99 |
Traditional High-Pressure Hydrogenation Route
Benzoic Acid Hydrogenation
Older methods hydrogenate benzoic acid to cyclohexanecarboxylic acid under extreme conditions (200–300°C, 50–100 bar H₂), often requiring nickel or ruthenium catalysts. This step generates by-products like cyclohexane and cyclohexanol, necessitating costly distillation or crystallization for purification.
Friedel-Crafts Acylation Challenges
Following chlorination, the Friedel-Crafts reaction traditionally uses benzene and AlCl₃ but faces limitations:
- Solvent Contamination : Residual solvents from prior steps (e.g., cyclohexane) necessitate removal before acylation.
- Low Atom Economy : Excess benzene (3–5 equivalents) is required to drive the reaction, increasing waste.
Manganate-Catalyzed Coupling Method
Reaction Overview
UK Patents 1,030,003 and 1,063,268 describe a single-step coupling of cyclohexanecarboxylic acid with benzoic acid using manganese carbonate (MnCO₃) at 280–450°C. While bypassing chlorination, this method suffers from:
- Thermal Degradation : High temperatures decompose reactants, reducing yields to <70%.
- Catalyst Deactivation : MnCO₃ forms oxides under oxidative conditions, requiring frequent replacement.
Solvent Selection and Process Design
Role of Benzene in Diels-Alder Synthesis
Benzene serves dual purposes:
- Reaction Medium : Facilitates the Diels-Alder step via π-π interactions with dienophiles.
- Friedel-Crafts Reactant : Eliminates solvent-switching steps, reducing processing time.
Non-Aromatic Alternatives
Hexane or cyclohexane can replace benzene in the Diels-Alder step, but subsequent benzene addition becomes necessary for acylation. This complicates the process compared to the integrated benzene approach.
Analytical Validation
Spectroscopic Characterization
Post-reaction analysis employs:
Chemical Reactions Analysis
Types of Reactions: Cyclohexyl phenyl ketone undergoes various chemical reactions, including:
Oxidation: It can be oxidized to form cyclohexyl phenyl carboxylic acid.
Reduction: Reduction of this compound can yield cyclohexyl phenyl alcohol.
Substitution: It can participate in nucleophilic substitution reactions, particularly at the carbonyl carbon.
Common Reagents and Conditions:
Oxidation: Common oxidizing agents include potassium permanganate and chromium trioxide.
Reduction: Sodium borohydride or lithium aluminum hydride are typically used as reducing agents.
Substitution: Reagents such as Grignard reagents can be used for nucleophilic substitution reactions.
Major Products:
Oxidation: Cyclohexyl phenyl carboxylic acid.
Reduction: Cyclohexyl phenyl alcohol.
Substitution: Various substituted cyclohexyl phenyl derivatives depending on the nucleophile used.
Scientific Research Applications
Chemical Properties and Safety
- Chemical Formula : C₁₃H₁₆O
- Molecular Weight : 188.26 g/mol
- CAS Number : 712-50-5
- Melting Point : 55-57 °C
- Hazard Classification : Irritant (Xi), toxic to aquatic life with long-lasting effects .
Intermediate in Synthesis
This compound serves as an intermediate in the synthesis of various organic compounds, including:
- Photosensitizers : It is utilized in the production of photoinitiators for polymerization processes, particularly in UV-curable coatings and inks .
- Medicinal Chemistry : This compound is a precursor for synthesizing antispasmodic agents like cyclohexyl phenyl methanol, which is crucial in pharmaceutical formulations .
Leather Treatment
This compound is employed as an intermediate in leather treatment processes. It enhances the durability and aesthetic properties of leather products, making it valuable in the leather industry .
Biocatalysis Studies
Recent studies have highlighted the use of Lactobacillus paracasei BD101 as a biocatalyst for the reduction of this compound to its corresponding alcohol. This process demonstrates high enantioselectivity and conversion rates, showcasing its potential in green chemistry applications .
Catalysis Research
This compound has been investigated as a substrate in metal-organic framework-supported catalysis for hydroboration and hydroamination reactions. These studies indicate its utility in facilitating complex organic transformations under mild conditions .
Case Study 1: Synthesis Optimization
A study focused on optimizing the synthesis of this compound from a mixture of hexahydrobenzoic acid and benzoic acid demonstrated high yields (up to 98.9%) through careful control of reaction conditions. The research emphasized the efficiency of using hydrochloric acid as a catalyst in this process, which maintained high purity levels of the final product .
Case Study 2: Photoinitiator Development
In developing new photoinitiators for UV curing applications, this compound was incorporated into formulations that exhibited improved curing rates and material properties. This application underscores its role in advancing materials science, particularly for coatings that require rapid curing under UV light .
Summary Table of Applications
Application Area | Description | Key Benefits |
---|---|---|
Synthesis Intermediate | Used in the production of photosensitizers and pharmaceuticals | Enhances efficiency in synthesis |
Leather Treatment | Improves durability and aesthetics of leather products | Increases product lifespan |
Biocatalysis | Reduces this compound to alcohol using bacteria | Promotes green chemistry practices |
Catalysis Research | Serves as a substrate for various catalytic reactions | Enables complex organic transformations |
Mechanism of Action
Cyclohexyl phenyl ketone exerts its effects primarily through its interaction with cellular components. For instance, it induces photohaemolysis in human erythrocytes upon exposure to ultraviolet A-rich irradiation. This process involves the absorption of UV light, leading to the generation of reactive oxygen species that cause cell membrane damage .
Comparison with Similar Compounds
Structural Analogs and Physical Properties
Compound | Molecular Formula | Boiling Point (°C) | Stability (250°C, 5.5 days) | Key Functional Group |
---|---|---|---|---|
Cyclohexyl phenyl ketone | C₁₃H₁₆O | 164–166 (Tboilr) | Stable (no rearrangement) | Ketone |
Benzophenone | C₁₃H₁₀O | 305 | N/A | Ketone |
Cyclohexyl phenyl ether | C₁₂H₁₄O | N/A | Hydrolyzes/rearranges | Ether |
Cyclohexyl phenyl sulfide | C₁₂H₁₄S | N/A | Hydrolyzes/rearranges | Sulfide |
Dicyclohexyl ketone | C₁₃H₂₂O | N/A | N/A | Ketone |
Notes:
- This compound exhibits superior thermal stability compared to ether/sulfide analogs under high-temperature conditions (250°C) .
- Benzophenone, a dehydrogenation product of this compound, has a higher boiling point (305°C vs. 164–166°C) due to extended conjugation .
Reactivity in Catalytic Reactions
Steric Effects :
- Bulky substituents (e.g., cyclohexyl, tert-butyl) reduce catalytic activity. For example, tertiary butyl phenyl ketone fails to undergo reduction with Mn(I) catalysts, whereas this compound shows moderate reactivity .
Functional Group Transformations
- Dehydrogenation: this compound undergoes aromatization to benzophenone under Cu catalysis, while dialkyl ketones (e.g., cyclohexanone) form alkenes or phenols .
- Electrochemical Degradation : α-Chlorinated derivatives show 72.3% COD removal efficiency at 100 mA/cm², following zero-order kinetics. The BOD₅/COD ratio improves from 0.22 to 0.46, enhancing biodegradability .
Biological Activity
Cyclohexyl phenyl ketone (CHPK) is a compound of significant interest in various fields, including organic chemistry, pharmacology, and materials science. Its unique structure, featuring a cyclohexyl group attached to a phenyl ketone, lends it diverse biological activities and applications. This article explores the biological activity of CHPK, including its synthesis, mechanisms of action, and case studies highlighting its effects.
Chemical Structure and Properties
This compound has the molecular formula and features a carbonyl group () bonded to both a cyclohexyl and a phenyl group. The cyclohexyl ring adopts a chair conformation, which influences its reactivity and interactions with biological systems .
Property | Value |
---|---|
Molecular Weight | 196.27 g/mol |
Melting Point | Not specified |
Boiling Point | Not specified |
Solubility | Soluble in organic solvents |
This compound exhibits various biological activities, primarily attributed to its structural characteristics. It acts as a photoinitiator in polymerization processes due to its ability to generate free radicals upon exposure to UV light. This property is utilized in the production of coatings, adhesives, and dental materials .
In addition to its photoinitiating capabilities, CHPK has been implicated in allergic reactions. A case study reported instances of photoallergic contact dermatitis associated with exposure to CHPK and related compounds, indicating potential sensitization effects in individuals previously exposed to similar ketones .
Case Studies
- Photoinitiator Activity : Research demonstrated that CHPK is effective as a photoinitiator in acrylic polymerization. The efficiency of CHPK was compared with other initiators, showing favorable results in terms of curing speed and final product properties .
- Allergic Reactions : A clinical study highlighted cases of allergic contact dermatitis linked to CHPK exposure in printing inks. Patients exhibited reactions upon contact with products containing this compound, suggesting the need for regulatory attention regarding its use in consumer products .
Table 2: Biological Activities and Effects
Activity | Description | References |
---|---|---|
Photoinitiator | Initiates polymerization under UV light | |
Allergic Contact Dermatitis | Associated with sensitization in exposed individuals |
Synthesis Methods
The synthesis of this compound typically involves Friedel-Crafts acylation reactions. One notable method employs cyclohexanecarbonyl chloride reacted with phenol in the presence of aluminum chloride as a catalyst. This method yields high selectivity and conversion rates, making it suitable for industrial applications .
Table 3: Synthesis Overview
Method | Reactants | Yield |
---|---|---|
Friedel-Crafts Acylation | Cyclohexanecarbonyl chloride + Phenol | >99% |
Q & A
Basic Research Questions
Q. What are the common synthetic routes for cyclohexyl phenyl ketone in laboratory settings?
this compound is synthesized via Friedel-Crafts acylation, where cyclohexane derivatives react with benzoyl chloride in the presence of Lewis acids like AlCl₃ . Alternative methods include oxidation of α-hydroxythis compound using phase-transfer catalysts (e.g., carbon tetrachloride and sodium hydroxide) under controlled conditions . Recent optimizations involve one-step procedures with improved yields (~85%) by adjusting solvent polarity and reaction temperature .
Q. What analytical techniques are recommended for characterizing this compound purity and structure?
High-purity samples (≥99%) are validated using HPLC with UV detection (λ = 254 nm) and chiral columns for enantiomeric separation . Structural confirmation employs H/C NMR, IR (C=O stretch ~1700 cm⁻¹), and mass spectrometry (m/z 204.27 for [M+H]⁺) . Purity criteria include moisture (<0.1%) and ash content (<0.5%) via Karl Fischer titration and gravimetric analysis .
Q. How is this compound utilized as a photoinitiator in UV-curable systems?
As a Type I photoinitiator (e.g., Irgacure 184), it undergoes Norrish Type I cleavage under UV light (λ = 250–350 nm), generating free radicals to initiate polymerization. Key parameters include UV intensity (10–50 mW/cm²) and catalyst loading (1–5 wt%) . Stability studies recommend storage in dark, inert conditions to prevent premature degradation .
Advanced Research Questions
Q. How does cyclohexyl ring strain influence reaction kinetics with sodium borohydride?
Kinetic studies (0–35°C) reveal reduced hydride affinity due to cyclohexyl ring strain, lowering reaction rates compared to cyclopentyl analogs. At 0°C, this compound exhibits a relative rate constant of 0.25 (vs. acetophenone = 1.0), attributed to torsional strain destabilizing the transition state . Conformational analysis via DFT suggests planar alignment of the ketone carbonyl and cyclohexyl ring optimizes steric hindrance, impacting reactivity .
Q. What methodological challenges arise in asymmetric hydrosilylation of this compound?
Bulky substituents on the cyclohexyl group reduce enantioselectivity (e.g., 83% ee vs. 86% for ethyl phenyl ketone) due to steric clashes with chiral catalysts like trans-diaminocyclohexane complexes. Optimization requires ligand screening (e.g., macrocycle L18 vs. acyclic L8) and silane stoichiometry (1.2 equiv.) in non-polar solvents (toluene, RT) .
Q. How do conflicting data on migratory aptitudes in thermal rearrangements inform mechanistic studies?
During pyrolysis (200–220°C), phenyl migration dominates over alkyl groups (4d yield >90%), but steric bulk at the migration origin can invert trends. For example, pentyl groups exhibit higher migratory aptitude than phenyl in strained systems, as shown by NMR and computational models . Contradictions highlight the need for context-dependent analysis of steric/electronic factors .
Q. What strategies resolve contradictions in Baeyer-Villiger oxidation mechanisms involving this compound?
Quantum mechanical studies (B3LYP/6-311+G(d,p)) identify acid-catalyzed Criegee intermediate formation as rate-limiting (ΔG‡ ≈ 38–41 kcal/mol). While cyclohexyl groups slow migration due to steric hindrance, strong acids (e.g., H₂SO₄) accelerate both addition and migration steps, reconciling divergent kinetic data .
Properties
IUPAC Name |
cyclohexyl(phenyl)methanone | |
---|---|---|
Source | PubChem | |
URL | https://pubchem.ncbi.nlm.nih.gov | |
Description | Data deposited in or computed by PubChem | |
InChI |
InChI=1S/C13H16O/c14-13(11-7-3-1-4-8-11)12-9-5-2-6-10-12/h1,3-4,7-8,12H,2,5-6,9-10H2 | |
Source | PubChem | |
URL | https://pubchem.ncbi.nlm.nih.gov | |
Description | Data deposited in or computed by PubChem | |
InChI Key |
BMFYCFSWWDXEPB-UHFFFAOYSA-N | |
Source | PubChem | |
URL | https://pubchem.ncbi.nlm.nih.gov | |
Description | Data deposited in or computed by PubChem | |
Canonical SMILES |
C1CCC(CC1)C(=O)C2=CC=CC=C2 | |
Source | PubChem | |
URL | https://pubchem.ncbi.nlm.nih.gov | |
Description | Data deposited in or computed by PubChem | |
Molecular Formula |
C13H16O | |
Source | PubChem | |
URL | https://pubchem.ncbi.nlm.nih.gov | |
Description | Data deposited in or computed by PubChem | |
DSSTOX Substance ID |
DTXSID5048182 | |
Record name | Cyclohexylphenylketone | |
Source | EPA DSSTox | |
URL | https://comptox.epa.gov/dashboard/DTXSID5048182 | |
Description | DSSTox provides a high quality public chemistry resource for supporting improved predictive toxicology. | |
Molecular Weight |
188.26 g/mol | |
Source | PubChem | |
URL | https://pubchem.ncbi.nlm.nih.gov | |
Description | Data deposited in or computed by PubChem | |
CAS No. |
712-50-5 | |
Record name | Cyclohexyl phenyl ketone | |
Source | CAS Common Chemistry | |
URL | https://commonchemistry.cas.org/detail?cas_rn=712-50-5 | |
Description | CAS Common Chemistry is an open community resource for accessing chemical information. Nearly 500,000 chemical substances from CAS REGISTRY cover areas of community interest, including common and frequently regulated chemicals, and those relevant to high school and undergraduate chemistry classes. This chemical information, curated by our expert scientists, is provided in alignment with our mission as a division of the American Chemical Society. | |
Explanation | The data from CAS Common Chemistry is provided under a CC-BY-NC 4.0 license, unless otherwise stated. | |
Record name | Cyclohexylphenylketone | |
Source | ChemIDplus | |
URL | https://pubchem.ncbi.nlm.nih.gov/substance/?source=chemidplus&sourceid=0000712505 | |
Description | ChemIDplus is a free, web search system that provides access to the structure and nomenclature authority files used for the identification of chemical substances cited in National Library of Medicine (NLM) databases, including the TOXNET system. | |
Record name | Cyclohexyl phenyl ketone | |
Source | DTP/NCI | |
URL | https://dtp.cancer.gov/dtpstandard/servlet/dwindex?searchtype=NSC&outputformat=html&searchlist=818 | |
Description | The NCI Development Therapeutics Program (DTP) provides services and resources to the academic and private-sector research communities worldwide to facilitate the discovery and development of new cancer therapeutic agents. | |
Explanation | Unless otherwise indicated, all text within NCI products is free of copyright and may be reused without our permission. Credit the National Cancer Institute as the source. | |
Record name | Methanone, cyclohexylphenyl- | |
Source | EPA Chemicals under the TSCA | |
URL | https://www.epa.gov/chemicals-under-tsca | |
Description | EPA Chemicals under the Toxic Substances Control Act (TSCA) collection contains information on chemicals and their regulations under TSCA, including non-confidential content from the TSCA Chemical Substance Inventory and Chemical Data Reporting. | |
Record name | Cyclohexylphenylketone | |
Source | EPA DSSTox | |
URL | https://comptox.epa.gov/dashboard/DTXSID5048182 | |
Description | DSSTox provides a high quality public chemistry resource for supporting improved predictive toxicology. | |
Record name | Cyclohexyl phenyl ketone | |
Source | European Chemicals Agency (ECHA) | |
URL | https://echa.europa.eu/substance-information/-/substanceinfo/100.010.840 | |
Description | The European Chemicals Agency (ECHA) is an agency of the European Union which is the driving force among regulatory authorities in implementing the EU's groundbreaking chemicals legislation for the benefit of human health and the environment as well as for innovation and competitiveness. | |
Explanation | Use of the information, documents and data from the ECHA website is subject to the terms and conditions of this Legal Notice, and subject to other binding limitations provided for under applicable law, the information, documents and data made available on the ECHA website may be reproduced, distributed and/or used, totally or in part, for non-commercial purposes provided that ECHA is acknowledged as the source: "Source: European Chemicals Agency, http://echa.europa.eu/". Such acknowledgement must be included in each copy of the material. ECHA permits and encourages organisations and individuals to create links to the ECHA website under the following cumulative conditions: Links can only be made to webpages that provide a link to the Legal Notice page. | |
Record name | CYCLOHEXYLPHENYLKETONE | |
Source | FDA Global Substance Registration System (GSRS) | |
URL | https://gsrs.ncats.nih.gov/ginas/app/beta/substances/0NU53987V8 | |
Description | The FDA Global Substance Registration System (GSRS) enables the efficient and accurate exchange of information on what substances are in regulated products. Instead of relying on names, which vary across regulatory domains, countries, and regions, the GSRS knowledge base makes it possible for substances to be defined by standardized, scientific descriptions. | |
Explanation | Unless otherwise noted, the contents of the FDA website (www.fda.gov), both text and graphics, are not copyrighted. They are in the public domain and may be republished, reprinted and otherwise used freely by anyone without the need to obtain permission from FDA. Credit to the U.S. Food and Drug Administration as the source is appreciated but not required. | |
Synthesis routes and methods I
Procedure details
Synthesis routes and methods II
Procedure details
Retrosynthesis Analysis
AI-Powered Synthesis Planning: Our tool employs the Template_relevance Pistachio, Template_relevance Bkms_metabolic, Template_relevance Pistachio_ringbreaker, Template_relevance Reaxys, Template_relevance Reaxys_biocatalysis model, leveraging a vast database of chemical reactions to predict feasible synthetic routes.
One-Step Synthesis Focus: Specifically designed for one-step synthesis, it provides concise and direct routes for your target compounds, streamlining the synthesis process.
Accurate Predictions: Utilizing the extensive PISTACHIO, BKMS_METABOLIC, PISTACHIO_RINGBREAKER, REAXYS, REAXYS_BIOCATALYSIS database, our tool offers high-accuracy predictions, reflecting the latest in chemical research and data.
Strategy Settings
Precursor scoring | Relevance Heuristic |
---|---|
Min. plausibility | 0.01 |
Model | Template_relevance |
Template Set | Pistachio/Bkms_metabolic/Pistachio_ringbreaker/Reaxys/Reaxys_biocatalysis |
Top-N result to add to graph | 6 |
Feasible Synthetic Routes
Disclaimer and Information on In-Vitro Research Products
Please be aware that all articles and product information presented on BenchChem are intended solely for informational purposes. The products available for purchase on BenchChem are specifically designed for in-vitro studies, which are conducted outside of living organisms. In-vitro studies, derived from the Latin term "in glass," involve experiments performed in controlled laboratory settings using cells or tissues. It is important to note that these products are not categorized as medicines or drugs, and they have not received approval from the FDA for the prevention, treatment, or cure of any medical condition, ailment, or disease. We must emphasize that any form of bodily introduction of these products into humans or animals is strictly prohibited by law. It is essential to adhere to these guidelines to ensure compliance with legal and ethical standards in research and experimentation.