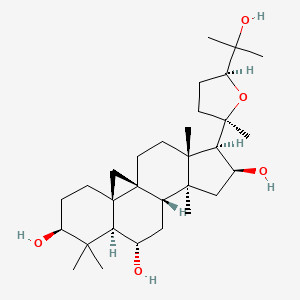
Cycloastragenol
Overview
Description
Mechanism of Action
Mode of Action
Cycloastragenol interacts with its targets to induce significant changes. It activates telomerase, leading to the elongation of telomeres . This process decreases the percentage of critically short telomeres and DNA damage in the cell . In addition, this compound binds to cathepsin B, inhibiting the lysosomal degradation of MHC-I and promoting the aggregation of MHC-I to the cell membrane . This boosts the presentation of the tumor antigen .
Biochemical Pathways
This compound affects several biochemical pathways. It is associated with the inhibition of Bcl-2 antiapoptotic family proteins and the PI3K/AKT/mTOR pathway . These pathways are involved in cell survival and proliferation, and their inhibition leads to the induction of apoptosis in senescent cells . Additionally, this compound has been found to regulate oxidative stress, neurotrophic processes, neuroinflammation, and apoptotic cell death .
Pharmacokinetics
This compound is absorbed through the intestinal epithelium via passive diffusion and undergoes first-pass hepatic metabolism . Compared with astragaloside IV, this compound is more lipid-soluble and more easily absorbed transdermally . Encapsulation of this compound in phospholipid vesicles enhances the transport and delivery across the skin barrier, making it more bioavailable for transdermal absorption .
Result of Action
The molecular and cellular effects of this compound’s action are profound. It selectively kills senescent cells by inducing apoptosis . This process is associated with the inhibition of Bcl-2 antiapoptotic family proteins and the PI3K/AKT/mTOR pathway . This compound treatment also suppresses the development of the senescence-associated secretory phenotype (SASP) in senescent cells, thereby inhibiting cell migration mediated by the SASP .
Action Environment
The action, efficacy, and stability of this compound can be influenced by environmental factors. For instance, the preparation of this compound can be carried out by hydrolysis of astragaloside IV using three methods: acid hydrolysis, enzymatic hydrolysis, and Smith degradation . The choice of method can affect the yield and purity of this compound. Furthermore, the bioavailability of this compound can be enhanced by encapsulating it in phospholipid vesicles, which can be a practical approach to make it more bioavailable for transdermal absorption in different environmental conditions .
Biochemical Analysis
Biochemical Properties
Cycloastragenol plays a vital role in biochemical reactions, particularly in the regulation of oxidative stress and neuroinflammation. It interacts with several enzymes and proteins, including brain-derived neurotrophic factor (BDNF), tropomyosin receptor kinase B (TrkB), nuclear factor erythroid 2–related factor 2 (Nrf2), and heme oxygenase-1 (HO-1) . This compound enhances the expression of BDNF and the phosphorylation of TrkB, which are crucial for neurogenesis and neuronal survival. Additionally, it upregulates Nrf2 and HO-1, which are involved in the cellular defense against oxidative stress .
Cellular Effects
This compound exerts various effects on different cell types and cellular processes. It has been shown to influence cell signaling pathways, gene expression, and cellular metabolism. In neuronal cells, this compound reduces oxidative stress and neuroinflammation, thereby protecting against neurodegenerative conditions . It also enhances the expression of neurogenic markers such as BDNF and NeuN (neuronal nuclear protein), which are essential for neuronal function and survival . In plant cells, this compound activates the telomere/telomerase system, leading to increased transcript levels of positive regulators of telomere maintenance .
Molecular Mechanism
The molecular mechanism of this compound involves its interaction with various biomolecules and its ability to modulate gene expression. This compound binds to and activates telomerase, leading to the elongation of telomeres and the maintenance of genomic stability . It also interacts with signaling pathways such as the mitogen-activated protein kinase (MAPK) pathway, where it downregulates the expression of phospho c-Jun-N-terminal kinase (p-JNK), p-38, and phospho-extracellular signal-related kinase (ERK1/2), thereby reducing oxidative stress and inflammation .
Temporal Effects in Laboratory Settings
In laboratory settings, the effects of this compound have been observed to change over time. This compound is relatively stable and can exert long-term effects on cellular function. In in vitro studies, this compound has been shown to maintain its activity over extended periods, leading to sustained upregulation of neurogenic markers and reduction of oxidative stress . In in vivo studies, this compound has demonstrated long-term neuroprotective effects in animal models of neurodegenerative diseases .
Dosage Effects in Animal Models
The effects of this compound vary with different dosages in animal models. At lower doses, this compound has been shown to activate telomerase and enhance neurogenesis without causing adverse effects . At higher doses, this compound may exhibit toxic effects, including hepatotoxicity and nephrotoxicity . It is essential to determine the optimal dosage to maximize the beneficial effects while minimizing potential toxicity.
Metabolic Pathways
This compound is involved in several metabolic pathways, including those related to oxidative stress and inflammation. It interacts with enzymes such as Nrf2 and HO-1, which play critical roles in the cellular defense against oxidative damage . This compound also influences metabolic flux and metabolite levels by modulating the expression of genes involved in telomere maintenance and neuroprotection .
Transport and Distribution
This compound is transported and distributed within cells and tissues through various mechanisms. It interacts with transporters and binding proteins that facilitate its uptake and localization within specific cellular compartments . This compound accumulates in the brain, where it exerts its neuroprotective effects by enhancing the expression of neurogenic markers and reducing oxidative stress .
Subcellular Localization
The subcellular localization of this compound is crucial for its activity and function. This compound is primarily localized in the cytoplasm and nucleus, where it interacts with telomerase and other biomolecules involved in telomere maintenance and neuroprotection . Post-translational modifications and targeting signals may direct this compound to specific compartments or organelles, enhancing its efficacy and stability .
Preparation Methods
Astramembrangenin can be synthesized through the hydrolysis of astragaloside IV, a glycoside found in Astragalus membranaceus. There are three primary methods for this hydrolysis:
Acid Hydrolysis: This method involves using an acid to break down astragaloside IV into Astramembrangenin.
Enzymatic Hydrolysis: Enzymes are used to catalyze the hydrolysis process, offering a more controlled and specific reaction.
Smith Degradation: This method involves the oxidative cleavage of glycosidic bonds, followed by reduction.
Chemical Reactions Analysis
Astramembrangenin undergoes various chemical reactions, including:
Oxidation: It can be oxidized to form different derivatives.
Reduction: Reduction reactions can modify its functional groups.
Substitution: Various substituents can be introduced to its structure through substitution reactions.
Common reagents used in these reactions include oxidizing agents like potassium permanganate and reducing agents like sodium borohydride. The major products formed depend on the specific reaction conditions and reagents used .
Scientific Research Applications
Astramembrangenin has a wide range of scientific research applications:
Chemistry: It is used in studies related to telomerase activation and its potential to extend telomeres.
Biology: Research has shown its effects on cellular senescence and its ability to promote cell proliferation.
Medicine: It is being explored for its anti-aging, anti-inflammatory, and cardiovascular protective effects.
Industry: Its potential use in anti-aging products and supplements is being investigated
Comparison with Similar Compounds
Astramembrangenin is unique due to its potent telomerase activation activity. Similar compounds include:
Astragaloside IV: The precursor to Astramembrangenin, known for its similar but less potent effects.
Cyclogalegigenin: Another triterpenoid with similar properties but different bioavailability.
Cyclosieversigenin: A compound with comparable chemical structure and effects .
Astramembrangenin stands out due to its higher bioavailability and more potent telomerase activation compared to these similar compounds.
Biological Activity
Cycloastragenol (CAG) is a natural compound derived from Astragalus membranaceus, a herb used in traditional Chinese medicine. It has garnered attention for its diverse biological activities, particularly in the fields of hepatoprotection, anti-fibrotic effects, telomerase activation, and neuroprotection. This article synthesizes recent findings on the biological activity of this compound, supported by case studies and detailed research data.
1. Hepatoprotective Effects
This compound has shown significant hepatoprotective effects in various studies, particularly against liver fibrosis induced by carbon tetrachloride (CCl₄) in animal models.
Key Findings:
- Dosage and Efficacy : In a study where mice were treated with CCl₄, administration of CAG at a dosage of 200 mg/kg resulted in reduced collagen deposition and downregulation of mRNA expression of collagen types I and III, indicating its anti-fibrotic efficacy .
- Mechanisms of Action : CAG enhanced the expression of matrix metalloproteinases (Mmps), which are crucial for collagen degradation. Specifically, Mmp8, proMmp9, and Mmp12 levels were significantly increased, contributing to fibrosis resolution .
- Histological Analysis : Histological assessments revealed that CAG treatment mitigated liver damage as evidenced by decreased serum alanine aminotransferase levels and preserved liver architecture .
Data Table: Hepatoprotective Efficacy of this compound
Parameter | Control (CCl₄) | CAG (200 mg/kg) | Significance |
---|---|---|---|
Serum ALT Levels (U/L) | 150 ± 10 | 80 ± 5 | p < 0.01 |
Collagen Deposition (µg/g) | 300 ± 20 | 150 ± 15 | p < 0.01 |
Mmp8 Expression (Relative Units) | 1.0 | 2.5 | p < 0.001 |
2. Telomerase Activation
Recent research highlights the role of this compound as a telomerase activator, which may have implications for reproductive biology and aging.
Study Insights :
- In Vitro Maturation : In bovine oocyte maturation studies, CAG significantly improved maturation rates (90.87%) and embryo cleavage rates (90.78%) compared to controls .
- Mechanistic Pathways : The activation of telomerase was associated with enhanced mitochondrial function, as indicated by increased mitochondrial membrane potential in treated groups .
3. Neuroprotective Properties
This compound has also been studied for its neuroprotective effects, particularly in promoting axon regeneration following spinal cord injuries.
Research Highlights :
- Axon Regeneration : A study demonstrated that CAG administration promoted axon growth in dorsal root ganglion neurons after spinal cord injury. The length of axons was significantly greater in CAG-treated mice compared to controls .
- Cell Survival : CAG did not adversely affect neuronal survival during culture conditions, suggesting its potential as a therapeutic agent for nerve injuries .
4. Anti-inflammatory and Anti-apoptotic Activities
This compound exhibits significant anti-inflammatory properties that contribute to its protective effects in various disease models.
Case Study :
Properties
IUPAC Name |
(1S,3R,6S,8R,9S,11S,12S,14S,15R,16R)-15-[(2R,5S)-5-(2-hydroxypropan-2-yl)-2-methyloxolan-2-yl]-7,7,12,16-tetramethylpentacyclo[9.7.0.01,3.03,8.012,16]octadecane-6,9,14-triol | |
---|---|---|
Source | PubChem | |
URL | https://pubchem.ncbi.nlm.nih.gov | |
Description | Data deposited in or computed by PubChem | |
InChI |
InChI=1S/C30H50O5/c1-24(2)20(33)8-11-30-16-29(30)13-12-26(5)23(28(7)10-9-21(35-28)25(3,4)34)18(32)15-27(26,6)19(29)14-17(31)22(24)30/h17-23,31-34H,8-16H2,1-7H3/t17-,18-,19-,20-,21-,22-,23-,26+,27-,28+,29-,30+/m0/s1 | |
Source | PubChem | |
URL | https://pubchem.ncbi.nlm.nih.gov | |
Description | Data deposited in or computed by PubChem | |
InChI Key |
WENNXORDXYGDTP-UOUCMYEWSA-N | |
Source | PubChem | |
URL | https://pubchem.ncbi.nlm.nih.gov | |
Description | Data deposited in or computed by PubChem | |
Canonical SMILES |
CC1(C(CCC23C1C(CC4C2(C3)CCC5(C4(CC(C5C6(CCC(O6)C(C)(C)O)C)O)C)C)O)O)C | |
Source | PubChem | |
URL | https://pubchem.ncbi.nlm.nih.gov | |
Description | Data deposited in or computed by PubChem | |
Isomeric SMILES |
C[C@]12CC[C@@]34C[C@@]35CC[C@@H](C([C@@H]5[C@H](C[C@H]4[C@@]1(C[C@@H]([C@@H]2[C@]6(CC[C@H](O6)C(C)(C)O)C)O)C)O)(C)C)O | |
Source | PubChem | |
URL | https://pubchem.ncbi.nlm.nih.gov | |
Description | Data deposited in or computed by PubChem | |
Molecular Formula |
C30H50O5 | |
Source | PubChem | |
URL | https://pubchem.ncbi.nlm.nih.gov | |
Description | Data deposited in or computed by PubChem | |
DSSTOX Substance ID |
DTXSID301029686 | |
Record name | (3beta,6alpha,9beta,16beta,20R,24S)-20,24-Epoxy-9,19-cyclolanostane-3,6,16,25-tetrol | |
Source | EPA DSSTox | |
URL | https://comptox.epa.gov/dashboard/DTXSID301029686 | |
Description | DSSTox provides a high quality public chemistry resource for supporting improved predictive toxicology. | |
Molecular Weight |
490.7 g/mol | |
Source | PubChem | |
URL | https://pubchem.ncbi.nlm.nih.gov | |
Description | Data deposited in or computed by PubChem | |
CAS No. |
78574-94-4, 84605-18-5 | |
Record name | Cycloastragenol | |
Source | CAS Common Chemistry | |
URL | https://commonchemistry.cas.org/detail?cas_rn=78574-94-4 | |
Description | CAS Common Chemistry is an open community resource for accessing chemical information. Nearly 500,000 chemical substances from CAS REGISTRY cover areas of community interest, including common and frequently regulated chemicals, and those relevant to high school and undergraduate chemistry classes. This chemical information, curated by our expert scientists, is provided in alignment with our mission as a division of the American Chemical Society. | |
Explanation | The data from CAS Common Chemistry is provided under a CC-BY-NC 4.0 license, unless otherwise stated. | |
Record name | Cycloastragenol | |
Source | ChemIDplus | |
URL | https://pubchem.ncbi.nlm.nih.gov/substance/?source=chemidplus&sourceid=0078574944 | |
Description | ChemIDplus is a free, web search system that provides access to the structure and nomenclature authority files used for the identification of chemical substances cited in National Library of Medicine (NLM) databases, including the TOXNET system. | |
Record name | (3beta,6alpha,9beta,16beta,20R,24S)-20,24-Epoxy-9,19-cyclolanostane-3,6,16,25-tetrol | |
Source | EPA DSSTox | |
URL | https://comptox.epa.gov/dashboard/DTXSID301029686 | |
Description | DSSTox provides a high quality public chemistry resource for supporting improved predictive toxicology. | |
Record name | (3β,6α,16β,24R)-20,24-Epoxy-9,19-cyclolanostane-3,6,16,25-tetrol | |
Source | European Chemicals Agency (ECHA) | |
URL | https://echa.europa.eu/information-on-chemicals | |
Description | The European Chemicals Agency (ECHA) is an agency of the European Union which is the driving force among regulatory authorities in implementing the EU's groundbreaking chemicals legislation for the benefit of human health and the environment as well as for innovation and competitiveness. | |
Explanation | Use of the information, documents and data from the ECHA website is subject to the terms and conditions of this Legal Notice, and subject to other binding limitations provided for under applicable law, the information, documents and data made available on the ECHA website may be reproduced, distributed and/or used, totally or in part, for non-commercial purposes provided that ECHA is acknowledged as the source: "Source: European Chemicals Agency, http://echa.europa.eu/". Such acknowledgement must be included in each copy of the material. ECHA permits and encourages organisations and individuals to create links to the ECHA website under the following cumulative conditions: Links can only be made to webpages that provide a link to the Legal Notice page. | |
Record name | CYCLOASTRAGENOL | |
Source | FDA Global Substance Registration System (GSRS) | |
URL | https://gsrs.ncats.nih.gov/ginas/app/beta/substances/X37D9F2L0V | |
Description | The FDA Global Substance Registration System (GSRS) enables the efficient and accurate exchange of information on what substances are in regulated products. Instead of relying on names, which vary across regulatory domains, countries, and regions, the GSRS knowledge base makes it possible for substances to be defined by standardized, scientific descriptions. | |
Explanation | Unless otherwise noted, the contents of the FDA website (www.fda.gov), both text and graphics, are not copyrighted. They are in the public domain and may be republished, reprinted and otherwise used freely by anyone without the need to obtain permission from FDA. Credit to the U.S. Food and Drug Administration as the source is appreciated but not required. | |
Retrosynthesis Analysis
AI-Powered Synthesis Planning: Our tool employs the Template_relevance Pistachio, Template_relevance Bkms_metabolic, Template_relevance Pistachio_ringbreaker, Template_relevance Reaxys, Template_relevance Reaxys_biocatalysis model, leveraging a vast database of chemical reactions to predict feasible synthetic routes.
One-Step Synthesis Focus: Specifically designed for one-step synthesis, it provides concise and direct routes for your target compounds, streamlining the synthesis process.
Accurate Predictions: Utilizing the extensive PISTACHIO, BKMS_METABOLIC, PISTACHIO_RINGBREAKER, REAXYS, REAXYS_BIOCATALYSIS database, our tool offers high-accuracy predictions, reflecting the latest in chemical research and data.
Strategy Settings
Precursor scoring | Relevance Heuristic |
---|---|
Min. plausibility | 0.01 |
Model | Template_relevance |
Template Set | Pistachio/Bkms_metabolic/Pistachio_ringbreaker/Reaxys/Reaxys_biocatalysis |
Top-N result to add to graph | 6 |
Feasible Synthetic Routes
Disclaimer and Information on In-Vitro Research Products
Please be aware that all articles and product information presented on BenchChem are intended solely for informational purposes. The products available for purchase on BenchChem are specifically designed for in-vitro studies, which are conducted outside of living organisms. In-vitro studies, derived from the Latin term "in glass," involve experiments performed in controlled laboratory settings using cells or tissues. It is important to note that these products are not categorized as medicines or drugs, and they have not received approval from the FDA for the prevention, treatment, or cure of any medical condition, ailment, or disease. We must emphasize that any form of bodily introduction of these products into humans or animals is strictly prohibited by law. It is essential to adhere to these guidelines to ensure compliance with legal and ethical standards in research and experimentation.