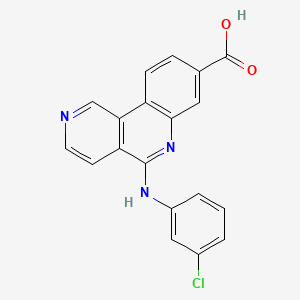
Silmitasertib
Overview
Description
Mechanism of Action
Target of Action
Silmitasertib, also known as CX-4945, is a small-molecule inhibitor of protein kinase CK2 . Protein kinase CK2 is a constitutively active serine/threonine-specific protein kinase that is overexpressed in several types of tumors . It is involved in the regulation of a plethora of biological processes and basal cellular functions, including growth, proliferation, and differentiation .
Mode of Action
This compound interacts competitively with the ATP-binding site of CK2 subunit alpha . This interaction leads to the inhibition of CK2, thereby disrupting its normal function .
Biochemical Pathways
The inhibition of CK2 by this compound leads to the disruption of several downstream signaling pathways, including PI3K/Akt . CK2 is involved in the regulation of several signaling pathways that are important for innate immune responses . Therefore, the inhibition of CK2 can have a significant impact on these pathways.
Pharmacokinetics
This compound has been found to have satisfactory bioavailability when given orally . , which is important for drug metabolism.
Result of Action
The inhibition of CK2 by this compound has been shown to have anti-cancer effects. It promotes cell cycle arrest and induces caspase activity and apoptosis in various cancer cell lines . In addition, this compound has been found to display potent antiviral activity in SARS-CoV-2 (COVID-19) infected cells .
Action Environment
The efficacy of this compound can be influenced by various environmental factors. For instance, the presence of other drugs can affect the bioavailability and efficacy of this compound. Furthermore, the specific cellular environment, such as the presence of certain proteins or the state of the immune system, can also influence the action of this compound .
Biochemical Analysis
Biochemical Properties
Silmitasertib interacts competitively with the ATP-binding site of the CK2 subunit alpha . By inhibiting CK2, this compound disrupts several downstream signaling pathways, including the PI3K/Akt pathway . CK2 is involved in the regulation of numerous cellular processes, such as cell growth, proliferation, and survival . This compound’s inhibition of CK2 leads to the suppression of these processes, making it an effective anti-cancer agent.
Cellular Effects
This compound has been shown to induce apoptosis and autophagy in various cancer cell lines, including pancreatic cancer cells and non-small cell lung cancer cells . It also promotes macropinocytosis in cholangiocarcinoma and colorectal cancer cells . By inhibiting CK2, this compound affects cell signaling pathways, gene expression, and cellular metabolism, leading to reduced cell proliferation and increased cell death .
Molecular Mechanism
At the molecular level, this compound exerts its effects by binding to the ATP-binding site of CK2 subunit alpha . This competitive inhibition prevents CK2 from phosphorylating its substrates, leading to the disruption of several signaling pathways, including PI3K/Akt . The inhibition of CK2 by this compound also results in the degradation of MCL1, a protein that promotes cell survival . This degradation sensitizes cancer cells to apoptosis, enhancing the compound’s anti-cancer effects .
Temporal Effects in Laboratory Settings
In laboratory settings, this compound has demonstrated stability and sustained activity over time . Long-term studies have shown that this compound can enhance the effects of other chemotherapeutic agents, such as vinblastine and doxorubicin, by facilitating their uptake within cells . This synergistic effect helps overcome chemoresistance, a common issue in long-term cancer treatment .
Dosage Effects in Animal Models
In animal models, this compound has shown dose-dependent effects on tumor growth . At higher doses, this compound effectively inhibits tumor growth and induces apoptosis without causing significant toxicity . At very high doses, some adverse effects, such as weight loss and mild organ toxicity, have been observed . These findings highlight the importance of optimizing dosage to maximize therapeutic benefits while minimizing side effects.
Metabolic Pathways
This compound is involved in several metabolic pathways, primarily through its inhibition of CK2 . CK2 is known to regulate various metabolic processes, including glucose uptake and amino acid synthesis . By inhibiting CK2, this compound can alter metabolic flux and metabolite levels, leading to changes in cellular metabolism that contribute to its anti-cancer effects .
Transport and Distribution
This compound is orally bioavailable and can be effectively transported and distributed within cells and tissues . It interacts with various transporters and binding proteins that facilitate its uptake and accumulation in target cells . This efficient transport and distribution are crucial for this compound’s therapeutic efficacy.
Subcellular Localization
This compound primarily localizes to the cytoplasm, where it inhibits CK2 activity . It has also been shown to affect the localization of other proteins, such as mTORC1, to specific subcellular compartments . These changes in subcellular localization can influence the activity and function of this compound, contributing to its overall therapeutic effects.
Preparation Methods
Synthetic Routes and Reaction Conditions: Silmitasertib is synthesized through a series of chemical reactions starting from benzo[c][2,6]naphthyridine-8-carboxylic acid. . The reaction conditions typically involve the use of organic solvents and catalysts to facilitate the formation of the desired product.
Industrial Production Methods: The industrial production of this compound involves scaling up the synthetic routes used in laboratory settings. This includes optimizing reaction conditions to ensure high yield and purity of the final product. Techniques such as liquid-liquid extraction and chromatography are employed to purify the compound .
Chemical Reactions Analysis
Types of Reactions: Silmitasertib undergoes various chemical reactions, including:
Oxidation: The compound can be oxidized under specific conditions to form oxidized derivatives.
Reduction: Reduction reactions can be used to modify the functional groups present in this compound.
Common Reagents and Conditions:
Oxidation: Common oxidizing agents include potassium permanganate and hydrogen peroxide.
Reduction: Reducing agents such as sodium borohydride and lithium aluminum hydride are used.
Substitution: Reagents like halogens and nucleophiles are used under controlled conditions to achieve substitution reactions.
Major Products Formed: The major products formed from these reactions depend on the specific reagents and conditions used. For example, oxidation may yield hydroxylated derivatives, while substitution reactions can introduce various functional groups onto the aromatic ring .
Scientific Research Applications
Silmitasertib has a wide range of scientific research applications, including:
Comparison with Similar Compounds
TWS119: Another CK2 inhibitor with a different chemical structure but similar inhibitory effects on CK2.
Quercetin: A natural flavonoid that also inhibits CK2 but with lower potency compared to Silmitasertib.
Myricetin: Similar to Quercetin, it is a natural compound with CK2 inhibitory activity.
Uniqueness of this compound: this compound stands out due to its high potency and selectivity for CK2. It has a favorable pharmacokinetic profile, including oral bioavailability and a long half-life, making it suitable for clinical use . Additionally, its ability to synergize with other anti-cancer agents enhances its therapeutic potential .
Biological Activity
Silmitasertib, also known as CX-4945, is a small-molecule inhibitor primarily targeting casein kinase 2 (CK2), a serine/threonine kinase involved in various cellular processes, including cell proliferation, survival, and differentiation. This compound has shown promising biological activity across multiple cancer types and other diseases, making it a significant focus of ongoing research.
This compound inhibits CK2, which is often overexpressed in various cancers. By blocking CK2 activity, this compound disrupts several signaling pathways that promote tumor growth and survival. Notably, it has been shown to:
- Inhibit pro-survival signaling : this compound effectively reduces the viability of cancer cells by interfering with their survival pathways. For example, studies demonstrated that it inhibits pro-survival and angiogenic signaling in breast, leukemia, colon, lung, and prostate cancer cell lines .
- Induce cell cycle arrest : In colorectal cancer (CRC) cells, this compound has been shown to induce G2/M arrest by decreasing mTOR activity, which is crucial for cell cycle progression .
- Promote methuosis-like cell death : Research indicates that CK2 inhibition leads to a specific type of cell death distinct from apoptosis, contributing to its anti-cancer effects .
Table 1: In Vitro Effects of this compound on Cancer Cell Lines
In Vivo Studies
In vivo studies have demonstrated the efficacy of this compound in various murine models. For instance:
- Breast and Pancreatic Cancer Models : this compound exhibited significant anti-tumor activity in murine xenograft models, leading to reduced tumor growth and improved survival rates .
- Combination Therapies : In clinical trials involving patients with locally advanced or metastatic cholangiocarcinoma (CCA), this compound combined with gemcitabine and cisplatin showed promising results with a median overall survival of 17.4 months .
Clinical Trials
This compound is currently being evaluated in various clinical trials for its safety and efficacy:
- Phase I/II Trials : Initial results from trials involving patients with recurrent SHH medulloblastoma indicated that this compound effectively inhibits the CK2 pathway with manageable side effects .
- COVID-19 Studies : Recent trials have explored its potential in treating moderate COVID-19 cases, showing statistically significant improvements in clinical recovery times .
Case Studies
- Cholangiocarcinoma : A study involving 87 patients treated with this compound in combination with standard chemotherapy reported an overall response rate of 32.1% and a disease control rate of 79.3% .
- Oral Submucous Fibrosis : Research indicated that this compound could inhibit TGF-β1 signaling, which is implicated in the malignant transformation associated with oral submucous fibrosis (OSMF) .
Side Effects and Safety Profile
While this compound has shown considerable promise as an anti-cancer agent, it is not without side effects. Common treatment-related adverse events include:
- Diarrhea (65.5%)
- Nausea (50.6%)
- Fatigue (31.0%)
Most adverse events were manageable, but some patients discontinued treatment due to severe reactions such as neutropenia and thrombocytopenia .
Properties
IUPAC Name |
5-(3-chloroanilino)benzo[c][2,6]naphthyridine-8-carboxylic acid | |
---|---|---|
Source | PubChem | |
URL | https://pubchem.ncbi.nlm.nih.gov | |
Description | Data deposited in or computed by PubChem | |
InChI |
InChI=1S/C19H12ClN3O2/c20-12-2-1-3-13(9-12)22-18-15-6-7-21-10-16(15)14-5-4-11(19(24)25)8-17(14)23-18/h1-10H,(H,22,23)(H,24,25) | |
Source | PubChem | |
URL | https://pubchem.ncbi.nlm.nih.gov | |
Description | Data deposited in or computed by PubChem | |
InChI Key |
MUOKSQABCJCOPU-UHFFFAOYSA-N | |
Source | PubChem | |
URL | https://pubchem.ncbi.nlm.nih.gov | |
Description | Data deposited in or computed by PubChem | |
Canonical SMILES |
C1=CC(=CC(=C1)Cl)NC2=NC3=C(C=CC(=C3)C(=O)O)C4=C2C=CN=C4 | |
Source | PubChem | |
URL | https://pubchem.ncbi.nlm.nih.gov | |
Description | Data deposited in or computed by PubChem | |
Molecular Formula |
C19H12ClN3O2 | |
Source | PubChem | |
URL | https://pubchem.ncbi.nlm.nih.gov | |
Description | Data deposited in or computed by PubChem | |
DSSTOX Substance ID |
DTXSID40143602 | |
Record name | Silmitasertib | |
Source | EPA DSSTox | |
URL | https://comptox.epa.gov/dashboard/DTXSID40143602 | |
Description | DSSTox provides a high quality public chemistry resource for supporting improved predictive toxicology. | |
Molecular Weight |
349.8 g/mol | |
Source | PubChem | |
URL | https://pubchem.ncbi.nlm.nih.gov | |
Description | Data deposited in or computed by PubChem | |
CAS No. |
1009820-21-6 | |
Record name | Silmitasertib [INN] | |
Source | ChemIDplus | |
URL | https://pubchem.ncbi.nlm.nih.gov/substance/?source=chemidplus&sourceid=1009820216 | |
Description | ChemIDplus is a free, web search system that provides access to the structure and nomenclature authority files used for the identification of chemical substances cited in National Library of Medicine (NLM) databases, including the TOXNET system. | |
Record name | Silmitasertib | |
Source | DrugBank | |
URL | https://www.drugbank.ca/drugs/DB15408 | |
Description | The DrugBank database is a unique bioinformatics and cheminformatics resource that combines detailed drug (i.e. chemical, pharmacological and pharmaceutical) data with comprehensive drug target (i.e. sequence, structure, and pathway) information. | |
Explanation | Creative Common's Attribution-NonCommercial 4.0 International License (http://creativecommons.org/licenses/by-nc/4.0/legalcode) | |
Record name | Silmitasertib | |
Source | EPA DSSTox | |
URL | https://comptox.epa.gov/dashboard/DTXSID40143602 | |
Description | DSSTox provides a high quality public chemistry resource for supporting improved predictive toxicology. | |
Record name | SILMITASERTIB | |
Source | FDA Global Substance Registration System (GSRS) | |
URL | https://gsrs.ncats.nih.gov/ginas/app/beta/substances/C6RWP0N0L2 | |
Description | The FDA Global Substance Registration System (GSRS) enables the efficient and accurate exchange of information on what substances are in regulated products. Instead of relying on names, which vary across regulatory domains, countries, and regions, the GSRS knowledge base makes it possible for substances to be defined by standardized, scientific descriptions. | |
Explanation | Unless otherwise noted, the contents of the FDA website (www.fda.gov), both text and graphics, are not copyrighted. They are in the public domain and may be republished, reprinted and otherwise used freely by anyone without the need to obtain permission from FDA. Credit to the U.S. Food and Drug Administration as the source is appreciated but not required. | |
Retrosynthesis Analysis
AI-Powered Synthesis Planning: Our tool employs the Template_relevance Pistachio, Template_relevance Bkms_metabolic, Template_relevance Pistachio_ringbreaker, Template_relevance Reaxys, Template_relevance Reaxys_biocatalysis model, leveraging a vast database of chemical reactions to predict feasible synthetic routes.
One-Step Synthesis Focus: Specifically designed for one-step synthesis, it provides concise and direct routes for your target compounds, streamlining the synthesis process.
Accurate Predictions: Utilizing the extensive PISTACHIO, BKMS_METABOLIC, PISTACHIO_RINGBREAKER, REAXYS, REAXYS_BIOCATALYSIS database, our tool offers high-accuracy predictions, reflecting the latest in chemical research and data.
Strategy Settings
Precursor scoring | Relevance Heuristic |
---|---|
Min. plausibility | 0.01 |
Model | Template_relevance |
Template Set | Pistachio/Bkms_metabolic/Pistachio_ringbreaker/Reaxys/Reaxys_biocatalysis |
Top-N result to add to graph | 6 |
Feasible Synthetic Routes
Q1: What is the primary target of Silmitasertib and how does it exert its effects?
A1: this compound is a potent and selective inhibitor of Casein Kinase 2 (CK2). [, , , , , , , ] It binds to the ATP-binding site of CK2, preventing the enzyme from phosphorylating its downstream targets. [, , , ] This inhibition disrupts multiple signaling pathways involved in cell growth, proliferation, survival, and metabolism. [, , , , , , , ]
Q2: What are the key downstream signaling pathways affected by this compound treatment?
A2: this compound's inhibition of CK2 affects several crucial signaling pathways, including PI3K-AKT-mTOR, JAK-STAT, NFκB, Wnt, and DNA repair pathways. [, , , , ] The specific effects on each pathway depend on the cell type and context.
Q3: Can you provide examples of how this compound's downstream effects impact cancer cells?
A3: In various cancer models, this compound has been shown to:
- Induce apoptosis through the activation of caspase-9/3 and upregulation of death receptor 4 (DR4). []
- Trigger autophagy-mediated cell death by dephosphorylating acetyl-CoA carboxylase (ACC). []
- Promote degradation of β-catenin, disrupting Wnt signaling and impairing tumor cell survival. []
- Impair lysosomal utilization, leading to cell death, particularly in KRAS mutant cancer cells. [, ]
- Downregulate the expression of stemness markers and cancer stem cell surface antigens, suggesting an impact on cancer stem cell survival and self-renewal. []
Q4: Besides cancer, are there other diseases where this compound's mechanism of action shows therapeutic potential?
A4: Yes, this compound's ability to inhibit CK2 has also shown promise in preclinical models of:
- Oral Submucous Fibrosis: Potentially by inhibiting Transforming Growth Factor Beta 1 (TGF-β1). []
- White Matter Stroke: By preserving oligodendrocytes, axons, and axonal mitochondria, leading to improved functional recovery. []
- COVID-19: Potentially by interfering with viral protein synthesis and egress. [, , ]
Q5: What is the molecular formula and weight of this compound?
A5: The molecular formula of this compound is C19H13ClN2O2, and its molecular weight is 336.77 g/mol. []
Q6: Is there any spectroscopic data available for this compound?
A6: While specific spectroscopic data wasn't detailed in the provided abstracts, various analytical techniques are used to characterize and quantify this compound, including LC-MS/MS. []
Q7: How do structural modifications of the this compound scaffold affect its activity and selectivity?
A7: Research on pyrazolo[1,5-a]pyrimidine derivatives, structurally similar to this compound, revealed that modifications like macrocyclization can influence CK2 inhibitory potency and selectivity. [] The presence of a polar carboxylic acid moiety, common to many CK2 inhibitors, was crucial for potency but might impact cellular activity. [] Further research explored 5-(3-chlorophenylamino)benzo[c][2,6]naphthyridine derivatives, identifying compounds with improved CK2 inhibitory activity and selectivity compared to this compound. []
Q8: What evidence supports this compound's efficacy in preclinical cancer models?
A8:
- Cholangiocarcinoma: this compound demonstrated synergistic effects with gemcitabine and cisplatin in preclinical models and Phase Ib/II clinical trials for cholangiocarcinoma, improving progression-free survival. [, , , , ]
- Acute Myeloid Leukemia: this compound showed potent anti-leukemia effects in HOX-driven AML models by downregulating HOX/MEIS expression. []
- Malignant Peripheral Nerve Sheath Tumors (MPNSTs): this compound reduced MPNST cell viability and slowed tumor growth in vivo, partly through destabilization of β-catenin. []
Q9: Has this compound shown efficacy in any in vivo models beyond cancer?
A9: Yes, this compound demonstrated efficacy in a mouse xenograft model of human B-cell acute lymphoblastic leukemia, particularly when combined with an acid ceramidase inhibitor. [] Additionally, in a mouse model of white matter stroke, this compound improved functional recovery and protected against white matter ischemic injury. []
Q10: Are there any clinical trials exploring this compound's therapeutic potential?
A10: Yes, this compound has been investigated in clinical trials for various cancers, including cholangiocarcinoma, medulloblastoma, and biliary tract cancer. [, , ] It has received orphan drug designation from the U.S. Food and Drug Administration (FDA) for some of these indications. [] Results from a Phase Ib/II study for cholangiocarcinoma showed promising efficacy. [, , ] Clinical trials are also underway to evaluate its potential in treating COVID-19. []
Q11: Are there any known mechanisms of resistance to this compound?
A11: While specific resistance mechanisms to this compound are not extensively discussed in the provided research, some studies hint at potential adaptive responses. For example, in B-cell acute lymphoblastic leukemia cells, this compound treatment increased the expression of both ceramide synthase 1 (CERS1) and acid ceramidase (AC). [] While increased CERS1 could promote cell death, the upregulation of AC might counteract this effect by breaking down ceramide. [] This highlights the potential for cancer cells to adapt to CK2 inhibition and the need for combinatorial treatment strategies.
Q12: What are the implications of this compound's interaction with other kinases?
A13: While this compound is a highly selective CK2 inhibitor, it has shown affinity towards other kinases, including DYRK1A and GSK3β. [, ] This off-target activity could present both opportunities and challenges, potentially influencing its efficacy and safety profile. [, ]
Q13: How does this compound's pharmacokinetic profile impact its potential therapeutic applications?
A14: Understanding this compound's absorption, distribution, metabolism, and excretion (ADME) is crucial for optimizing its therapeutic use. [, , , , , ] For instance, developing strategies to improve its bioavailability or target its delivery to specific tissues could enhance its efficacy and minimize potential side effects. [, , ]
Disclaimer and Information on In-Vitro Research Products
Please be aware that all articles and product information presented on BenchChem are intended solely for informational purposes. The products available for purchase on BenchChem are specifically designed for in-vitro studies, which are conducted outside of living organisms. In-vitro studies, derived from the Latin term "in glass," involve experiments performed in controlled laboratory settings using cells or tissues. It is important to note that these products are not categorized as medicines or drugs, and they have not received approval from the FDA for the prevention, treatment, or cure of any medical condition, ailment, or disease. We must emphasize that any form of bodily introduction of these products into humans or animals is strictly prohibited by law. It is essential to adhere to these guidelines to ensure compliance with legal and ethical standards in research and experimentation.