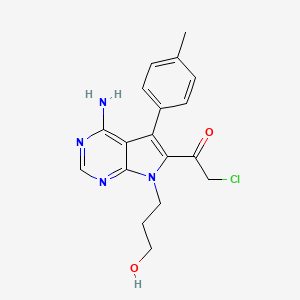
CMK
Overview
Description
The term "CMK" refers to distinct compounds across scientific disciplines, each with unique applications and properties. This article focuses on four primary contexts:
- Enzymatic CMKs: Cytidine monophosphate kinases involved in nucleotide metabolism.
- Carboxymethyl Konjac Glucomannan (this compound): A modified dietary fiber derived from konjac.
- Coal-Based Metakaolin (this compound): A construction material enhancing cement durability.
- This compound as a Furin Inhibitor: A compound inhibiting viral protease activity.
Each variant is compared to structurally or functionally similar compounds, supported by empirical data.
Preparation Methods
Synthesis of SBA-15 Silica Template
The structural fidelity of CMK-3 directly depends on the quality of the SBA-15 template. The patented method outlines a reproducible synthesis of SBA-15 using tetraethyl orthosilicate (TEOS) and Pluronic P123 surfactant under acidic conditions.
Reaction Conditions and Procedure
A mixture of distilled water (60.00 mL), 2 M hydrochloric acid (120.00 g), and Pluronic P123 (8.00 g) is stirred at 35°C until homogenized. TEOS (18.20 mL) is added dropwise, and the suspension is stirred at 35°C for 20 h. The precipitate is aged at 90°C for 24 h, washed, dried, and calcined at 550°C.
Table 1: SBA-15 Synthesis Parameters
Component | Quantity | Role |
---|---|---|
Pluronic P123 | 8.00 g | Structure-directing agent |
TEOS | 18.20 mL | Silica source |
HCl (2 M) | 120.00 g | Acid catalyst |
Calcination temperature | 550°C | Remove surfactant |
Simplified this compound-3 Synthesis Using Furfuryl Alcohol
The patented innovation streamlines this compound-3 production by eliminating solvent-intensive steps and pre-modification of SBA-15. Furfuryl alcohol serves as the carbon precursor, with hydrochloric acid catalyzing its polycondensation within SBA-15 pores.
Critical Reaction Parameters
- Mass Ratios :
SBA-15 : Water : Furfuryl Alcohol : HCl = 1.00 : 32.33–30.83 : 1.00–2.50 : 6.65–16.64. - Temperature : 30–100°C during polycondensation.
- Carbonization : 850°C under argon (4.6 purity, 40 mL/min) for 4 h.
Stepwise Procedure
- Slurry Preparation : Combine SBA-15 (3.00 g), furfuryl alcohol (6.00 g), water (94.00 mL), and HCl (34.10 mL).
- Polycondensation : Stir at 100°C for 6 h to form poly(furfuryl alcohol) (PFA)/SBA-15 composite.
- Carbonization : Heat to 850°C under argon to convert PFA to carbon.
- Template Removal : Etch silica with 5% hydrofluoric acid (2 × 90 min).
Table 2: Optimized this compound-3 Synthesis Conditions
Parameter | Value | Impact on Structure |
---|---|---|
HCl concentration | 35% (w/w) | Accelerates furfuryl alcohol polymerization |
Carbonization rate | 1°C/min | Prevents pore collapse |
HF treatment time | 180 min (total) | Complete silica removal |
Comparative Analysis of this compound-3 Synthesis Methods
The patented method addresses key limitations of earlier approaches:
Traditional Methods vs. Improved Protocol
The elimination of organic solvents reduces environmental and safety risks, while the aqueous slurry system enhances scalability.
Structural and Textural Properties of this compound-3
Nitrogen physisorption and TEM analyses confirm that the patented method yields this compound-3 with:
- Surface Area : 1,200–1,350 m²/g (BET method).
- Pore Volume : 1.5–1.7 cm³/g (mesopore-dominated).
- Pore Diameter : 4.2 nm (consistent with SBA-15 template).
Table 3: this compound-3 Structural Characteristics
Property | Value | Measurement Technique |
---|---|---|
Surface area | 1,300 ± 50 m²/g | BET |
Pore volume | 1.6 cm³/g | BJH adsorption |
Wall thickness | 3.8 nm | TEM |
Chemical Reactions Analysis
CMK materials undergo various chemical reactions, including oxidation, reduction, and substitution. For instance, this compound-3 can be modified with metal nanoparticles, such as iron oxide, to enhance its hydrogen storage capacity . The incorporation of metal species is typically achieved through wetness impregnation, where the metal precursor is introduced into the mesoporous carbon structure and subsequently reduced to form nanoparticles .
Common reagents used in these reactions include metal salts (e.g., iron nitrate) and reducing agents (e.g., sodium borohydride). The major products formed from these reactions are metal-modified this compound materials with enhanced catalytic or adsorption properties .
Scientific Research Applications
Oxidation Mechanisms
CMK serves as a valuable model compound for elucidating oxidation mechanisms in atmospheric chemistry. In a study conducted by Praplan et al., this compound was used to investigate gas-phase reactions involving hydroxyl radicals and ozone. The research revealed that this compound undergoes oxidation to produce several organic acids, including succinic acid and butyrolactone, which can be measured using ion chromatography coupled with mass spectrometry .
Key Findings:
- Reaction Pathways: The study identified various reaction pathways leading to the formation of primary oxidation products, highlighting the complexity of this compound's reactivity under different conditions.
- Experimental Conditions: The relative amount of this compound reacted varied significantly depending on the experimental setup, indicating that environmental factors play a crucial role in its oxidation behavior.
Neuroprotective Applications
This compound has also been investigated for its neuroprotective properties, particularly in the context of intracerebral hemorrhage (ICH). A study published by Lin et al. demonstrated that AC-YVAD-CMK, a selective inhibitor of caspase-1, could reduce pyroptosis—a form of inflammatory cell death—following ICH .
Case Study Insights:
- Mechanism of Action: The administration of AC-YVAD-CMK resulted in decreased activation of caspase-1 and reduced levels of interleukin-1 beta (IL-1β), suggesting a protective effect against neuroinflammation.
- Functional Recovery: Mice treated with AC-YVAD-CMK showed improved neurological function post-hemorrhage, indicating its potential as a therapeutic agent in neurodegenerative conditions.
Agricultural and Biobased Applications
Recent developments have explored the use of carboxymethyl-Kaumera (this compound), derived from natural sources, as a biobased material in agriculture. This new formulation is being researched for its properties as a biostimulant and binding agent .
Research Highlights:
- Properties and Applications: this compound exhibits binding, plasticizing, and fire-retardant properties, making it suitable for various agricultural applications.
- Ongoing Research: Current studies focus on optimizing production methods and scaling up for commercial use while assessing properties such as biodegradability and water retention capabilities.
Mechanism of Action
The mechanism of action of CMK materials depends on their specific application. In hydrogen storage, the high surface area and uniform pore size of this compound materials facilitate the physisorption of hydrogen molecules . When used as catalysts, the metal nanoparticles supported on this compound materials provide active sites for catalytic reactions, enhancing reaction rates and selectivity .
In biofuel cells, this compound-3 serves as a support for enzyme immobilization, improving the stability and activity of the enzymes involved in the electrochemical reactions . The mesoporous structure of this compound materials allows for efficient mass transport and electron transfer, contributing to the overall performance of the biofuel cell .
Comparison with Similar Compounds
Enzymatic CMKs: Structural and Functional Comparisons
Enzymatic CMKs phosphorylate cytidine monophosphate (CMP) or deoxycytidine monophosphate (dCMP) and are critical in nucleotide metabolism.
Substrate Specificity and Cofactor Usage
- Bifidobacterium CMK (Bithis compound) vs. E. coli this compound: Bithis compound exhibits similar catalytic efficiency for CMP and dCMP with ATP as the phosphate donor. However, E. coli this compound shows 2-fold higher activity for dCMP than CMP . Unlike E. coli this compound, Bithis compound’s activity decreases when GTP replaces ATP (e.g., 50% reduction for CMP phosphorylation with GTP) .
Structural Comparisons Across Species
- S. aureus this compound (Sathis compound) vs. S. pneumoniae this compound (Spthis compound) :
- E. coli this compound : Contains a unique sulfate ion in its active site, absent in Sathis compound, influencing inhibitor design .
Table 1: Catalytic and Structural Properties of Enzymatic CMKs
Carboxymethyl Konjac Glucomannan (this compound) vs. Konjac Glucomannan (KGM)
This compound is a chemically modified derivative of KGM, a natural polysaccharide.
Physicochemical Properties
- Solubility : this compound is water-soluble, unlike KGM, which requires alkaline conditions for dissolution .
- Viscosity : this compound has significantly lower viscosity (15.57 Pa·s) compared to KGM (typically >30 Pa·s) due to carboxymethyl group substitution .
- Ash Content : this compound’s ash content increases by 40% post-modification, indicating higher mineral retention .
This compound as a Furin Inhibitor vs. Other Antiviral Agents
This compound inhibits furin protease, a key enzyme in viral entry and replication.
Mechanism of Action
- SARS-CoV-2 : this compound reduces viral production by 50% (100 μM dose) and inhibits syncytium formation, while naphthofluorescein targets viral RNA transcription .
- HBV : this compound enhances HBV replication alone but, combined with entecavir, reduces viral load by 60% and HBeAg secretion .
Comparative Efficacy
- Calu-6 Lung Cancer Cells : this compound (100 μM) decreases cell proliferation by 50%, comparable to furin siRNA (60% reduction) .
Table 3: this compound vs. Other Furin Inhibitors
Agent | Target Pathway | Efficacy | Reference |
---|---|---|---|
This compound | Spike cleavage | 50% viral reduction | |
Naphthofluorescein | RNA transcription | 30% viral reduction | |
Furin siRNA | IGF-1R processing | 60% proliferation loss |
Coal-Based Metakaolin (this compound) vs. Traditional Cement Additives
This compound enhances cement’s resistance to chloride and sulfate corrosion.
Performance Metrics
- Surface Area : this compound (150 m²/g) has lower surface area than 3D graphene-like carbon (3DGC, 450 m²/g) but higher than activated carbon (AC, 100 m²/g) .
- Corrosion Resistance : this compound reduces cement expansion by 40% and increases compressive strength by 3-fold compared to unmodified cement .
- Optimal Ratio : A mix of 90% salt-affected soil, 2% cement, 6% S95 slag, and 2% this compound improves resistivity by 3.3-fold .
Table 4: this compound vs. Carbon Additives in Cement
Property | This compound | 3DGC | Activated Carbon |
---|---|---|---|
Surface Area (m²/g) | 150 | 450 | 100 |
Corrosion Expansion | 40% reduction | N/A | No significant effect |
Compressive Strength | 3x increase | N/A | 1.5x increase |
Gene Expression and Function
- Sequence Homology : Hbthis compound shares 72.6% similarity with Catharanthus roseus this compound, while Aathis compound shows 70% homology with Salvia miltiorrhiza .
- Induction: Aathis compound expression increases 8-fold under methyl jasmonate (MeJA) treatment, enhancing terpenoid production .
Table 5: this compound Gene Homology Across Plant Species
Biological Activity
Chlorocresol (CMK) is a compound with significant biological activity, primarily recognized for its antimicrobial properties. This article explores the diverse biological effects of this compound, including its efficacy against various pathogens, potential toxicity, and mechanisms of action, supported by relevant case studies and research findings.
1. Overview of this compound
This compound is a chlorinated cresol that serves as a biocide in various applications, including metalworking fluids and preservatives. It exhibits a broad spectrum of activity against bacteria and fungi, making it valuable in industrial and medical settings.
2. Antimicrobial Activity
2.1 Efficacy Against Bacteria and Fungi
This compound has demonstrated effectiveness against a range of microorganisms:
Microorganism | Efficacy | Concentration |
---|---|---|
Bacillus subtilis | Effective | 0.25-0.3% w/v |
Pseudomonas aeruginosa | Effective | 0.25-0.3% w/v |
Aspergillus niger | Effective | 1.2 g/L (0.12% w/w) |
Trichoderma viridae | Effective | 1.2 g/L (0.12% w/w) |
Studies indicate that this compound interacts with multiple targets within bacterial cell walls, reducing the likelihood of resistance development .
2.2 Resistance Development
Research shows that when used within recommended concentrations, this compound does not promote acquired resistance in microbial populations due to its multi-target action .
3. Toxicological Profile
3.1 Acute and Chronic Toxicity
Toxicological studies have assessed the safety profile of this compound:
- Acute Toxicity: Inhalation studies in Wistar rats indicated local effects at higher concentrations, with a NOAEL determined at 50 mg/m³ .
- Chronic Toxicity: Long-term dietary studies showed no adverse effects at doses up to 120 mg/kg bw/day for males and 170 mg/kg bw/day for females .
3.2 Endocrine Disruption Potential
While some studies suggest a slight potential for endocrine disruption in vitro, comprehensive assessments indicate no significant endocrine activity in vivo .
This compound's antimicrobial action is attributed to its ability to disrupt cellular processes in target organisms:
- Cell Membrane Disruption: this compound alters the permeability of microbial cell membranes, leading to cell lysis.
- Inhibition of Enzymatic Activity: It interferes with essential enzymes involved in metabolic pathways within bacteria and fungi.
5. Case Studies and Research Findings
Several studies highlight the biological activity of this compound:
- A study demonstrated that this compound effectively reduced microbial load in metalworking fluids, maintaining fluid integrity over extended periods .
- Another investigation into the antifungal properties of this compound reported successful inhibition of fungal growth in various industrial applications, confirming its utility as a preservative .
6. Conclusion
Chlorocresol (this compound) exhibits significant biological activity characterized by its antimicrobial efficacy against a variety of pathogens while maintaining a favorable safety profile under appropriate use conditions. Its multi-target mechanism reduces the risk of resistance development, making it an essential compound in both industrial and medical applications.
Q & A
Q. How can researchers optimize CMK dosage in catalytic systems for antibiotic degradation?
Basic Research Question
To determine the optimal this compound dosage in peroxydisulfate (PDS)-activated systems for sulfonamide antibiotic degradation, researchers should:
- Conduct parameter-controlled experiments varying this compound dosage (e.g., 0.05–0.2 g/L) while fixing other variables (e.g., PDS concentration, pH, temperature) .
- Measure degradation efficiency via high-performance liquid chromatography (HPLC) and track kinetic rates using pseudo-first-order models.
- Analyze trade-offs between cost (material usage) and efficiency. For instance, 0.2 g/L this compound achieves complete degradation within 5 minutes but may not be cost-effective for scaled applications .
Key Parameters to Monitor :
Variable | Range Tested | Optimal Value | Impact on Efficiency |
---|---|---|---|
This compound dosage | 0.05–0.2 g/L | 0.1 g/L | Linear increase |
Reaction time | 5–30 min | 15 min | Plateau after 15 min |
SDZ concentration | 10–50 mg/L | 20 mg/L | Inhibition at >30 mg/L |
Q. What advanced methods characterize the catalytic mechanism of this compound in PDS activation?
Advanced Research Question
Mechanistic studies require multi-technique characterization:
- Surface Analysis : Use Raman spectroscopy to identify defect sites and XPS to quantify oxygen-containing functional groups (e.g., C=O, O-C=O), which facilitate electron transfer in this compound/PDS systems .
- Radical Identification : Employ quenching experiments (e.g., methanol, tert-butanol) to distinguish between sulfate radicals (SO₄•⁻) and hydroxyl radicals (•OH).
- Non-Radical Pathways : Validate electron transfer via electrochemical impedance spectroscopy (EIS) and probe experiments with methyl phenyl sulfoxide (PMSO) .
Example Findings :
- This compound’s edge defects and oxygen groups enhance PDS activation.
- Both radical (SO₄•⁻, •OH) and non-radical (¹O₂, electron transfer) pathways contribute to SDZ degradation.
Q. How should researchers design experiments to evaluate this compound (Customer Master Key) rotation policies in cloud encryption?
Basic Research Question
To assess the impact of this compound rotation on data security:
- Simulate rotation cycles (e.g., 90-day intervals) using AWS KMS or Alibaba Cloud SDKs .
- Track decryption success rates for ciphertexts encrypted with prior key versions.
- Measure latency during key generation and re-encryption processes.
Methodological Steps :
Generate multiple this compound versions via KMS APIs.
Encrypt test datasets using each version.
Rotate keys and attempt decryption without specifying versions.
Analyze KMS’s automatic version detection accuracy .
Q. What hybrid encryption models integrate this compound with post-quantum algorithms for future-proofing research?
Advanced Research Question
For post-quantum security:
- Use this compound to generate symmetric data keys (AES-256) and pair them with lattice-based algorithms (e.g., Kyber) for key encapsulation .
- Benchmark performance (encryption/decryption speed, error rates) in hybrid setups using tools like OpenQuantumSafe.
- Validate compliance with NIST Post-Quantum Cryptography standards.
Example Workflow :
This compound → AES-256 (data encryption).
Kyber → Encapsulate AES key.
Store encapsulated key with ciphertext.
Q. How can this compound (machine capability index) assess reliability in automated harness equipment testing?
Basic Research Question
In engineering research:
- Define critical quality metrics (e.g., crimping stability, terminal profile accuracy) .
- Collect process data (n ≥ 50 samples) under controlled conditions.
- Calculate this compound as , where σ is short-term standard deviation.
Statistical Considerations :
- This compound > 1.67 indicates high process capability.
- Compare with Cpk (long-term capability) to identify systemic vs. random errors .
Q. How do researchers resolve contradictions between this compound and Cpk in process capability studies?
Advanced Research Question
Address discrepancies via:
- Root-Cause Analysis : Use ANOVA to partition variance (machine vs. operator effects).
- Design of Experiments (DoE) : Vary factors like temperature or pressure in factorial designs to isolate influences .
- Bayesian Methods : Update capability estimates dynamically with new data.
Case Study :
A this compound/Cpk mismatch in vascular burst pressure testing was resolved by identifying unaccounted material batch variability .
Properties
IUPAC Name |
1-[4-amino-7-(3-hydroxypropyl)-5-(4-methylphenyl)pyrrolo[2,3-d]pyrimidin-6-yl]-2-chloroethanone | |
---|---|---|
Source | PubChem | |
URL | https://pubchem.ncbi.nlm.nih.gov | |
Description | Data deposited in or computed by PubChem | |
InChI |
InChI=1S/C18H19ClN4O2/c1-11-3-5-12(6-4-11)14-15-17(20)21-10-22-18(15)23(7-2-8-24)16(14)13(25)9-19/h3-6,10,24H,2,7-9H2,1H3,(H2,20,21,22) | |
Source | PubChem | |
URL | https://pubchem.ncbi.nlm.nih.gov | |
Description | Data deposited in or computed by PubChem | |
InChI Key |
PELFTNQHGSITLB-UHFFFAOYSA-N | |
Source | PubChem | |
URL | https://pubchem.ncbi.nlm.nih.gov | |
Description | Data deposited in or computed by PubChem | |
Canonical SMILES |
CC1=CC=C(C=C1)C2=C(N(C3=NC=NC(=C23)N)CCCO)C(=O)CCl | |
Source | PubChem | |
URL | https://pubchem.ncbi.nlm.nih.gov | |
Description | Data deposited in or computed by PubChem | |
Molecular Formula |
C18H19ClN4O2 | |
Source | PubChem | |
URL | https://pubchem.ncbi.nlm.nih.gov | |
Description | Data deposited in or computed by PubChem | |
DSSTOX Substance ID |
DTXSID10586567 | |
Record name | 1-[4-Amino-7-(3-hydroxypropyl)-5-(4-methylphenyl)-7H-pyrrolo[2,3-d]pyrimidin-6-yl]-2-chloroethan-1-one | |
Source | EPA DSSTox | |
URL | https://comptox.epa.gov/dashboard/DTXSID10586567 | |
Description | DSSTox provides a high quality public chemistry resource for supporting improved predictive toxicology. | |
Molecular Weight |
358.8 g/mol | |
Source | PubChem | |
URL | https://pubchem.ncbi.nlm.nih.gov | |
Description | Data deposited in or computed by PubChem | |
CAS No. |
821794-90-5 | |
Record name | 1-[4-Amino-7-(3-hydroxypropyl)-5-(4-methylphenyl)-7H-pyrrolo[2,3-d]pyrimidin-6-yl]-2-chloroethan-1-one | |
Source | EPA DSSTox | |
URL | https://comptox.epa.gov/dashboard/DTXSID10586567 | |
Description | DSSTox provides a high quality public chemistry resource for supporting improved predictive toxicology. | |
Retrosynthesis Analysis
AI-Powered Synthesis Planning: Our tool employs the Template_relevance Pistachio, Template_relevance Bkms_metabolic, Template_relevance Pistachio_ringbreaker, Template_relevance Reaxys, Template_relevance Reaxys_biocatalysis model, leveraging a vast database of chemical reactions to predict feasible synthetic routes.
One-Step Synthesis Focus: Specifically designed for one-step synthesis, it provides concise and direct routes for your target compounds, streamlining the synthesis process.
Accurate Predictions: Utilizing the extensive PISTACHIO, BKMS_METABOLIC, PISTACHIO_RINGBREAKER, REAXYS, REAXYS_BIOCATALYSIS database, our tool offers high-accuracy predictions, reflecting the latest in chemical research and data.
Strategy Settings
Precursor scoring | Relevance Heuristic |
---|---|
Min. plausibility | 0.01 |
Model | Template_relevance |
Template Set | Pistachio/Bkms_metabolic/Pistachio_ringbreaker/Reaxys/Reaxys_biocatalysis |
Top-N result to add to graph | 6 |
Feasible Synthetic Routes
Disclaimer and Information on In-Vitro Research Products
Please be aware that all articles and product information presented on BenchChem are intended solely for informational purposes. The products available for purchase on BenchChem are specifically designed for in-vitro studies, which are conducted outside of living organisms. In-vitro studies, derived from the Latin term "in glass," involve experiments performed in controlled laboratory settings using cells or tissues. It is important to note that these products are not categorized as medicines or drugs, and they have not received approval from the FDA for the prevention, treatment, or cure of any medical condition, ailment, or disease. We must emphasize that any form of bodily introduction of these products into humans or animals is strictly prohibited by law. It is essential to adhere to these guidelines to ensure compliance with legal and ethical standards in research and experimentation.