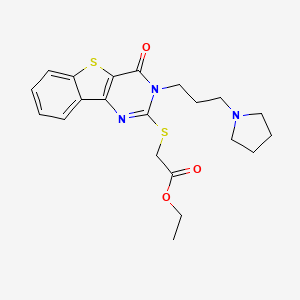
CM037
Overview
Description
The compound “A37” refers to a bacteriocin produced by the bacterium Staphylococcus epidermidis. Bacteriocins are antimicrobial peptides that inhibit the growth of other bacteria, providing a competitive advantage in natural environments. A37 is a member of the lantibiotic family, characterized by lanthionine groups as the defining structural motif .
Mechanism of Action
Target of Action
CM037, also known as Ethyl [4-Oxo-3-(3-pyrrolidin-1-yl-propyl)-3,4-dihydro-benzo[4,5]thieno[3,2-d]pyrimidin-2-ylsulfanyl]acetate or A-37, is a selective inhibitor of ALDH1A1 (aldehyde dehydrogenase 1A1) . ALDH1A1 is an enzyme that plays a crucial role in the oxidation of aldehydes to their corresponding carboxylic acids .
Mode of Action
This compound interacts with its target, ALDH1A1, by binding to the aldehyde binding pocket of the enzyme . This interaction inhibits the activity of ALDH1A1, preventing it from catalyzing the oxidation of aldehydes .
Biochemical Pathways
The inhibition of ALDH1A1 by this compound affects the aldehyde metabolism pathway. Under normal conditions, ALDH1A1 catalyzes the conversion of aldehydes, which are potentially toxic, into carboxylic acids. By inhibiting ALDH1A1, this compound prevents this conversion, leading to an accumulation of aldehydes .
Result of Action
The primary result of this compound’s action is the inhibition of ALDH1A1, leading to an accumulation of aldehydes. This can have various molecular and cellular effects, depending on the specific aldehydes that accumulate and their roles in cellular processes .
Action Environment
The action, efficacy, and stability of this compound can be influenced by various environmental factors. For instance, the pH of the environment can affect the compound’s solubility and therefore its bioavailability Additionally, the presence of other substances that can interact with ALDH1A1 or with this compound itself may also influence the compound’s action
Biochemical Analysis
Biochemical Properties
CM037 interacts with the enzyme ALDH1A1, inhibiting its activity . The inhibition of ALDH1A1 by this compound leads to an increase in the accumulation of aldehydes, a group of compounds that can be toxic to cells . This interaction is believed to be the primary mechanism by which this compound exerts its effects .
Cellular Effects
This compound has been shown to have significant effects on various types of cells. For example, it has been found to inhibit the growth of cancer cells by increasing the accumulation of toxic aldehydes .
Molecular Mechanism
The molecular mechanism of this compound involves its interaction with the enzyme ALDH1A1. This compound binds to the aldehyde binding pocket of ALDH1A1, inhibiting its activity . This leads to an increase in the accumulation of aldehydes, which can cause cellular damage and inhibit cell growth .
Temporal Effects in Laboratory Settings
Given its mechanism of action, it is likely that the effects of this compound would become more pronounced over time as the accumulation of aldehydes increases .
Dosage Effects in Animal Models
The effects of this compound at different dosages in animal models have not been extensively studied. It is known that this compound is a potent inhibitor of ALDH1A1, suggesting that even at low dosages, it could have significant effects on cellular function .
Metabolic Pathways
This compound is involved in the metabolic pathway of aldehyde oxidation. By inhibiting ALDH1A1, this compound prevents the conversion of aldehydes to their corresponding carboxylic acids .
Transport and Distribution
Given its small molecular size and its ability to pass through cell membranes, it is likely that this compound can be distributed throughout the body .
Subcellular Localization
Given that its target, ALDH1A1, is located in the cytoplasm of cells , it is likely that this compound also localizes to the cytoplasm where it can interact with ALDH1A1.
Preparation Methods
The preparation of bacteriocin A37 involves microbiological techniques. The bacterium Staphylococcus epidermidis is cultured under specific conditions to induce the production of A37. The compound is then isolated and purified using techniques such as chromatography and electrophoresis .
Chemical Reactions Analysis
Bacteriocin A37 undergoes various chemical reactions, including:
Oxidation: A37 can be oxidized under specific conditions, leading to the formation of disulfide bonds.
Reduction: Reduction reactions can break these disulfide bonds, altering the structure and function of A37.
Substitution: A37 can undergo substitution reactions where specific amino acids are replaced, potentially modifying its antimicrobial activity.
Scientific Research Applications
Bacteriocin A37 has several scientific research applications:
Chemistry: A37 is studied for its unique structural properties and potential as a model for designing new antimicrobial agents.
Biology: Research focuses on understanding the ecological role of A37 in microbial communities and its interactions with other bacteria.
Medicine: A37 is explored as a potential alternative to traditional antibiotics, particularly in treating infections caused by antibiotic-resistant bacteria.
Comparison with Similar Compounds
Bacteriocin A37 is unique compared to other lantibiotics due to its specific mode of action and ecological role. Similar compounds include:
Nisin: A well-known lantibiotic that targets the peptidoglycan synthesis pathway in bacteria.
Epilancin K7: Another lantibiotic with a different mode of action, primarily disrupting bacterial cell membranes.
Lacticin 3147: A lantibiotic with dual mechanisms of action, targeting both peptidoglycan synthesis and membrane integrity
Bacteriocin A37 stands out due to its specific uptake mechanism and the formation of intracellular vesicles, which are crucial for its antibacterial activity.
Properties
IUPAC Name |
ethyl 2-[[4-oxo-3-(3-pyrrolidin-1-ylpropyl)-[1]benzothiolo[3,2-d]pyrimidin-2-yl]sulfanyl]acetate | |
---|---|---|
Source | PubChem | |
URL | https://pubchem.ncbi.nlm.nih.gov | |
Description | Data deposited in or computed by PubChem | |
InChI |
InChI=1S/C21H25N3O3S2/c1-2-27-17(25)14-28-21-22-18-15-8-3-4-9-16(15)29-19(18)20(26)24(21)13-7-12-23-10-5-6-11-23/h3-4,8-9H,2,5-7,10-14H2,1H3 | |
Source | PubChem | |
URL | https://pubchem.ncbi.nlm.nih.gov | |
Description | Data deposited in or computed by PubChem | |
InChI Key |
SKDRHRAYBYQVNU-UHFFFAOYSA-N | |
Source | PubChem | |
URL | https://pubchem.ncbi.nlm.nih.gov | |
Description | Data deposited in or computed by PubChem | |
Canonical SMILES |
CCOC(=O)CSC1=NC2=C(C(=O)N1CCCN3CCCC3)SC4=CC=CC=C42 | |
Source | PubChem | |
URL | https://pubchem.ncbi.nlm.nih.gov | |
Description | Data deposited in or computed by PubChem | |
Molecular Formula |
C21H25N3O3S2 | |
Source | PubChem | |
URL | https://pubchem.ncbi.nlm.nih.gov | |
Description | Data deposited in or computed by PubChem | |
Molecular Weight |
431.6 g/mol | |
Source | PubChem | |
URL | https://pubchem.ncbi.nlm.nih.gov | |
Description | Data deposited in or computed by PubChem | |
Retrosynthesis Analysis
AI-Powered Synthesis Planning: Our tool employs the Template_relevance Pistachio, Template_relevance Bkms_metabolic, Template_relevance Pistachio_ringbreaker, Template_relevance Reaxys, Template_relevance Reaxys_biocatalysis model, leveraging a vast database of chemical reactions to predict feasible synthetic routes.
One-Step Synthesis Focus: Specifically designed for one-step synthesis, it provides concise and direct routes for your target compounds, streamlining the synthesis process.
Accurate Predictions: Utilizing the extensive PISTACHIO, BKMS_METABOLIC, PISTACHIO_RINGBREAKER, REAXYS, REAXYS_BIOCATALYSIS database, our tool offers high-accuracy predictions, reflecting the latest in chemical research and data.
Strategy Settings
Precursor scoring | Relevance Heuristic |
---|---|
Min. plausibility | 0.01 |
Model | Template_relevance |
Template Set | Pistachio/Bkms_metabolic/Pistachio_ringbreaker/Reaxys/Reaxys_biocatalysis |
Top-N result to add to graph | 6 |
Feasible Synthetic Routes
Q1: What makes CM037 a selective inhibitor of ALDH1A1?
A: this compound exhibits selectivity for human ALDH1A1 over eight other ALDH isoenzymes due to its unique binding interaction within the enzyme's active site. Crystal structure analysis reveals that this compound binds within the aldehyde binding pocket of ALDH1A1 and exploits the presence of a glycine residue unique to this isoform. This specific interaction contributes to its selectivity. []
Q2: How does this compound impact tumor angiogenesis in melanoma?
A: Research suggests that this compound, by inhibiting ALDH1A1, indirectly reduces the release of pro-angiogenic factors, specifically IL-8, from melanoma cells. This, in turn, downregulates the IL-8/Notch signaling cascade in endothelial cells, hindering their angiogenic activity. This effect was observed through reduced endothelial cell proliferation, migration, and tube formation in vitro, and reduced microvessel density in vivo. []
Q3: What are the structural characteristics of this compound?
A: this compound, chemically known as Ethyl ({4-Oxo-3-[3-(Pyrrolidin-1-Yl)propyl]-3,4-Dihydro[1]benzothieno[3,2-D]pyrimidin-2-Yl}sulfanyl)acetate, represents a distinct chemical class of ALDH1A1 inhibitors. [] Unfortunately, the provided research excerpts do not contain information about the molecular formula, weight, or spectroscopic data of this compound.
Q4: Has this compound shown synergy with other cancer treatments?
A: While not specifically mentioned for this compound, the research indicates that other ALDH inhibitors, such as 673A, demonstrate synergistic effects when combined with chemotherapy. This synergy results in enhanced tumor growth reduction. [] Further research is needed to investigate if this compound exhibits similar synergistic properties.
Disclaimer and Information on In-Vitro Research Products
Please be aware that all articles and product information presented on BenchChem are intended solely for informational purposes. The products available for purchase on BenchChem are specifically designed for in-vitro studies, which are conducted outside of living organisms. In-vitro studies, derived from the Latin term "in glass," involve experiments performed in controlled laboratory settings using cells or tissues. It is important to note that these products are not categorized as medicines or drugs, and they have not received approval from the FDA for the prevention, treatment, or cure of any medical condition, ailment, or disease. We must emphasize that any form of bodily introduction of these products into humans or animals is strictly prohibited by law. It is essential to adhere to these guidelines to ensure compliance with legal and ethical standards in research and experimentation.