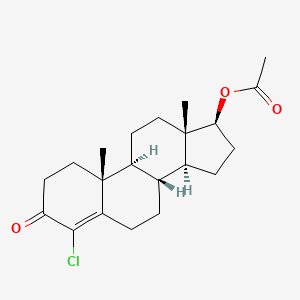
Clostebol acetate
Overview
Description
Clostebol acetate (C₂₁H₂₉ClO₃; molecular weight: 364.91 g/mol) is a synthetic anabolic-androgenic steroid (AAS) derived from testosterone via chlorination at the C4 position and esterification with an acetyl group at the 17β-hydroxyl position . Its systematic IUPAC name is (8R,9S,10R,13S,14S,17S)-4-chloro-3-oxoandrost-4-en-17-yl acetate . Structurally, the B and C rings of the steroidal backbone adopt chair conformations, while the A ring is a half-chair, and the D ring exists in a C13 envelope conformation .
This compound is primarily used in topical dermatological and ophthalmological preparations (e.g., Trofodermin®) for its anti-inflammatory and tissue-repair properties . However, its anabolic effects have led to widespread misuse in sports, prompting the World Anti-Doping Agency (WADA) to classify it as a prohibited substance in and out of competition .
Preparation Methods
Clostebol acetate’s synthesis revolves around the strategic introduction of a chlorine atom at the C4 position of the testosterone backbone, followed by esterification at the C17β hydroxyl group. Two primary routes dominate the literature: direct chlorination of testosterone acetate and epoxide-mediated chlorination . Both methods require precise control of stereochemistry to preserve the compound’s biological activity, as the absolute configurations at positions 8, 9, 10, 13, 14, and 17 are critical for receptor binding.
Direct Chlorination Method
Reaction Mechanism and Conditions
The direct chlorination approach involves treating testosterone acetate with chlorinating agents under controlled conditions. The reaction proceeds via electrophilic addition, where the Δ4 double bond in testosterone acetate reacts with a chlorine source to form the 4-chloro derivative.
Key reagents and conditions :
- Chlorinating agents : Thionyl chloride (SOCl₂), phosphorus pentachloride (PCl₅), or chlorine gas (Cl₂).
- Solvents : Dichloromethane, chloroform, or carbon tetrachloride.
- Temperature : 0–25°C to prevent over-chlorination or decomposition.
A representative reaction equation is:
$$
\text{Testosterone acetate} + \text{PCl}5 \xrightarrow{\text{CH}2\text{Cl}2, \, 0-5^\circ\text{C}} \text{this compound} + \text{POCl}3 + \text{HCl}
$$
Yield optimization : Early studies by Camerino et al. (1956) achieved yields of 68–72% using PCl₅ in anhydrous dichloromethane. Lower temperatures (0–5°C) minimized side products like 5α-chloro isomers.
Optimization of Chlorinating Agents
Industrial protocols favor thionyl chloride due to its liquid state and ease of handling. Comparative studies reveal:
Chlorinating Agent | Reaction Time (h) | Yield (%) | Purity (%) |
---|---|---|---|
PCl₅ | 4–6 | 72 | 95 |
SOCl₂ | 2–3 | 78 | 97 |
Cl₂ (gas) | 1–2 | 65 | 90 |
Thionyl chloride’s superior performance stems from its dual role as a solvent and reagent, facilitating faster kinetics and higher regioselectivity.
Epoxide Intermediate Method
Epoxidation of Testosterone Acetate
This two-step method first converts testosterone acetate to its 4,5-epoxide intermediate using peracids like meta-chloroperbenzoic acid (mCPBA):
$$
\text{Testosterone acetate} + \text{mCPBA} \xrightarrow{\text{CH}2\text{Cl}2, \, 25^\circ\text{C}} \text{4,5-Epoxy-testosterone acetate} + \text{mCBA}
$$
Conditions :
- Epoxidation : 12–24 hours at 20–25°C.
- Yield : 85–90% with <5% diastereomeric excess.
Ring-Opening with Hydrochloric Acid
The epoxide undergoes ring-opening with hydrochloric acid (HCl) to introduce the chlorine atom:
$$
\text{4,5-Epoxy-testosterone acetate} + \text{HCl} \xrightarrow{\text{H}2\text{O/THF}} \text{this compound} + \text{H}2\text{O}
$$
Optimization :
- Acid concentration : 6M HCl ensures complete ring-opening without ester hydrolysis.
- Temperature : 40–50°C accelerates the reaction (2–3 hours).
This method achieves 80–85% overall yield with higher stereochemical fidelity compared to direct chlorination.
Purification and Crystallization Techniques
Crude this compound is purified via selective crystallization. Methanol is the solvent of choice due to its polarity and temperature-dependent solubility:
Parameter | Value |
---|---|
Solvent | Methanol |
Temperature | 20–25°C (cooling) |
Crystallization time | 24–48 hours |
Purity post-crystallization | ≥99% |
Single-crystal X-ray diffraction confirms the orthorhombic crystal system (space group P2₁2₁2₁) with unit cell parameters:
$$
a = 7.740(1)\,Å, \, b = 12.631(2)\,Å, \, c = 19.275(2)\,Å, \, V = 1884.4(4)\,Å^3
$$
These crystallographic data ensure batch-to-batch consistency in pharmaceutical formulations.
Industrial-Scale Production Considerations
Large-scale synthesis prioritizes cost-efficiency and safety:
- Continuous flow reactors : Reduce reaction time by 40% compared to batch processes.
- In-line analytics : HPLC monitors chlorination efficiency in real-time.
- Waste management : HCl gas is scrubbed using NaOH solutions to minimize environmental impact.
Analytical Characterization of Synthetic Products
Quality control employs multiple techniques:
Method | Parameter Analyzed | Acceptance Criteria |
---|---|---|
HPLC-UV (λ=240 nm) | Purity | ≥98% area |
GC-MS | Residual solvents | <500 ppm |
XRD | Crystallinity | Match reference pattern |
Polarimetry | [α]D²⁵ (c=1, CHCl₃) | +102° to +108° |
The Flack parameter (x = -0.02(6)) confirms the correct absolute configuration.
Chemical Reactions Analysis
Types of Reactions: Clostebol acetate undergoes several types of chemical reactions, including:
Oxidation: this compound can be oxidized to form 4-chloroandrost-4-en-3,17-dione.
Reduction: Reduction of this compound can yield 4-chloroandrost-4-en-17β-ol-3-one.
Substitution: The chlorine atom at the 4-position can be substituted with other functional groups under appropriate conditions.
Common Reagents and Conditions:
Oxidation: Common oxidizing agents include potassium permanganate and chromium trioxide.
Reduction: Reducing agents such as lithium aluminum hydride or sodium borohydride are used.
Substitution: Nucleophilic substitution reactions can be carried out using reagents like sodium methoxide or sodium ethoxide.
Major Products Formed:
Oxidation: 4-chloroandrost-4-en-3,17-dione.
Reduction: 4-chloroandrost-4-en-17β-ol-3-one.
Substitution: Various substituted derivatives depending on the nucleophile used.
Scientific Research Applications
Medical Applications
1. Dermatological Uses
Clostebol acetate is commonly used in dermatology for its ability to promote healing and tissue regeneration. It is included in topical formulations aimed at treating:
- Wounds and Ulcers : this compound aids in the healing of various skin injuries, including abrasions, ulcers, and burns. It is often combined with other agents like neomycin in products such as Trofodermin®, which contains 5% this compound .
- Radiodermatitis : This condition arises from radiation therapy, leading to skin irritation. This compound helps alleviate symptoms by enhancing skin recovery .
2. Ophthalmological Uses
In ophthalmology, this compound has been utilized in treatments involving ocular conditions, although specific formulations are less commonly discussed compared to dermatological applications .
Veterinary Applications
This compound has been employed in veterinary medicine to enhance growth rates in livestock. Its anabolic properties facilitate improved muscle mass and overall growth in cattle, making it a subject of concern regarding animal welfare and food safety regulations .
Sports and Doping Concerns
This compound is classified as a prohibited substance by the World Anti-Doping Agency (WADA) due to its performance-enhancing effects. The following points outline significant findings related to its use in sports:
- Accidental Doping : Studies have indicated that athletes may test positive for clostebol metabolites due to accidental exposure from topical creams or contaminated meat. For instance, a case study highlighted an athlete who tested positive after using Trofodermin® on an injury .
- Transference Between Individuals : There are documented instances where clostebol was transferred between individuals through casual contact or sexual intercourse, leading to positive doping tests . This raises questions about the thresholds for detection and the need for clear guidelines regarding accidental exposure.
Detection Methods
The detection of clostebol metabolites in urine has evolved with advancements in analytical techniques:
- Gas Chromatography-Mass Spectrometry (GC-MS) : This method has been refined to improve sensitivity for detecting clostebol metabolites at lower concentrations .
- Liquid Chromatography-Mass Spectrometry (LC-MS) : LC-MS techniques have been employed to identify phase II metabolites of clostebol, enhancing the ability to determine the source of contamination or use .
Case Studies
Mechanism of Action
Clostebol acetate exerts its effects by binding to androgen receptors in the body. Once bound, the hormone-receptor complex enters the cell nucleus and binds to specific DNA sequences, activating the transcription of genes involved in protein synthesis. This leads to increased muscle growth and repair. The chlorination at the 4-position prevents conversion to dihydrotestosterone and estrogen, reducing the risk of androgenic and estrogenic side effects .
Comparison with Similar Compounds
Structural and Functional Differences
Clostebol Acetate vs. Testosterone
- Structural Modifications : this compound introduces a chlorine atom at C4 and an acetyl ester at C17β, reducing first-pass metabolism and enhancing oral bioavailability compared to unmodified testosterone .
This compound vs. Turinabol (4-Chlorodehydromethyltestosterone)
- Structural Similarities : Both compounds feature a C4 chlorine substitution. However, Turinabol includes an additional methyl group at C17α and a double bond between C1 and C2, enhancing its anabolic potency and resistance to hepatic breakdown .
- Metabolic Pathways: this compound is metabolized to 4-chlorotestosterone (C1) and diols (C3, C7), while Turinabol produces longer-lasting metabolites like 4-chloro-18-nor-17β-hydroxymethyl-17α-methyl-androsta-1,4,13-triene-3-one .
This compound vs. Anavar (Oxandrolone)
- Chemical Class : Anavar is a dihydrotestosterone (DHT) derivative with a 2-oxa substitution, whereas this compound retains the testosterone backbone.
- Anabolic-to-Androgenic Ratio : Anavar exhibits a higher anabolic ratio (3:1) compared to this compound (1:1), making it more selective for muscle growth with minimal virilization risks .
Pharmacokinetic and Detection Profiles
Table 1: Comparative Pharmacokinetics and Detection
Key Findings:
- Metabolic Stability: this compound’s acetyl group prolongs its half-life compared to non-esterified testosterone but remains shorter than Turinabol due to rapid deacetylation .
- Detection Sensitivity : this compound’s primary metabolite, 4-chloroandrost-4-ene-3α,17β-diol (C3), is detectable in urine at concentrations up to 30–40 ng/mL for 10 days post-exposure . Hair analysis can identify this compound at 1.1 pg/mg, making it a reliable long-term biomarker .
Abuse Potential and Contamination Risks
- Accidental Exposure : Transdermal application or contact with clostebol-containing creams (e.g., Trofodermin®) can lead to inadvertent doping violations, as even single exposures produce detectable urinary metabolites .
- Comparative Risk : Unlike Turinabol or Anavar, this compound’s widespread use in topical medications increases contamination risks, particularly in regions like Italy and Brazil .
Biological Activity
Clostebol acetate, a synthetic anabolic steroid derived from testosterone, has garnered significant attention in both clinical and sports contexts due to its biological activity and potential for misuse. This article explores the biological activity of this compound, focusing on its metabolism, routes of administration, detection in biological fluids, and implications for doping control.
Chemical Structure and Properties
This compound is characterized by its lipophilic nature, which allows it to penetrate biological membranes effectively. Its chemical structure includes a chlorine atom at the C4 position, which alters its metabolic pathways compared to other anabolic steroids. This modification affects its binding affinity to androgen receptors and its anabolic versus androgenic activity profile.
Metabolism and Excretion
The metabolism of this compound primarily occurs in the liver, leading to the formation of several metabolites. The main metabolite detected in urine is 4-chloro-androst-4-en-3α-ol-17-one (M1). Studies have shown that after transdermal application, M1 can be detected in urine for extended periods:
Metabolite | Detection Window | Concentration |
---|---|---|
M1 | Up to 25 days | 30-40 μg/L at peak |
Other Metabolites (M2-M4) | Varies | Generally lower concentrations |
The detection of these metabolites is crucial for anti-doping measures, as they can indicate recent use of this compound even after a single exposure .
Routes of Administration
This compound can be administered through various routes, including:
- Topical Application : Commonly used in formulations like Trofodermin®, this route allows for localized effects and systemic absorption. The drug diffuses through the skin layers and enters systemic circulation, which can lead to detectable levels in urine .
- Intravaginal Application : A study demonstrated that intravaginal application followed by sexual intercourse resulted in detectable levels of clostebol metabolites in male partners .
Case Studies and Incidents
Several case studies highlight the complexities surrounding this compound's use in sports:
- Accidental Doping : A male athlete tested positive for clostebol metabolites after sexual intercourse with a partner who had used a topical formulation. Urine samples showed trace amounts of M1 (0.9–3.5 μg/L), raising questions about incidental exposure versus intentional use .
- Contamination Cases : Reports have documented instances where athletes claimed contamination from handling animals treated with clostebol-containing products. For example, an athlete was found to have clostebol metabolites due to contact with a dog treated with Trofodermin® .
- Doping Control Challenges : The detection of clostebol metabolites poses challenges for anti-doping authorities. The potential for cross-contamination during intimate contact or through shared environments complicates the interpretation of positive test results .
Research Findings
Recent studies have focused on understanding the metabolic profiles of this compound:
- A study using liquid chromatography-tandem mass spectrometry identified fourteen metabolites of clostebol, including nine previously unreported ones. This research enhances the understanding of how long these metabolites remain detectable and their implications for doping control .
- Another study highlighted that glucuronide conjugates are primarily excreted metabolites, which can persist longer than others, providing valuable biomarkers for detecting misuse .
Q & A
Basic Research Questions
Q. What are the crystallographic characteristics of clostebol acetate, and how do they inform its molecular stability?
this compound crystallizes in an orthorhombic system (space group P2₁2₁2₁) with lattice parameters a = 7.740 Å, b = 12.631 Å, and c = 19.275 Å . Its absolute configuration (8R,9S,10R,13S,14S,17S) was confirmed via Flack parameter refinement. The steroid core adopts distinct conformations: chair (B, C rings), half-chair (A ring), and envelope (D ring). Weak C–H⋯O interactions stabilize the crystal lattice, forming fishbone-like chains along the b-axis . These structural insights are critical for understanding its solubility and interactions in biological systems.
Q. What is the synthetic pathway for this compound, and how is purity ensured?
this compound is synthesized by direct chlorination at the 4-position of testosterone acetate or via epoxidation followed by chlorination. The crude product is purified via selective crystallization from methanol, yielding colorless prisms . Purity is validated using X-ray diffraction (R[F² > 2σ(F²)] = 0.038, wR(F²) = 0.079) and HPLC with >98% assay .
Q. How does this compound exert its therapeutic effects in dermatological applications?
As a topical agent, this compound enhances wound healing by modulating collagen synthesis and reducing inflammation. Its low systemic absorption minimizes androgenic side effects, making it suitable for treating ulcers, burns, and radiation dermatitis . Preclinical studies in mice showed no significant toxicity on weight, hematology, or liver function at therapeutic doses .
Advanced Research Questions
Q. How can analytical methods be optimized for detecting this compound and its metabolites in anti-doping contexts?
Hair analysis provides a robust matrix for long-term detection. Intraday precision (CV%) ranges from 6–20% for clostebol and 9–14% for its acetate, with biases ≤9% across low (1 pg/mg), medium (25 pg/mg), and high (100 pg/mg) concentrations . Liquid chromatography-tandem mass spectrometry (LC-MS/MS) with multiple reaction monitoring (MRM) improves sensitivity, detecting metabolites like 4-chloro-androsterone at sub-ng/mL levels . Method optimization should prioritize reducing interday variability (e.g., 14–30% CV for clostebol) via internal standardization and matrix-matched calibration .
Q. What role do crystal packing interactions play in this compound’s bioavailability?
The crystal structure’s weak C–H⋯O interactions (2.5–3.0 Å) influence dissolution kinetics. These interactions reduce solubility in polar solvents, necessitating esterification (e.g., acetate) to enhance lipophilicity for transdermal delivery . Computational modeling of these interactions could guide prodrug design to improve pharmacokinetics.
Q. How does this compound alter metabolic pathways in fungal systems?
Metabolomic studies in fungi show this compound upregulates phospholipid derivatives (e.g., LPE 18:2, PE 18:2/0:0) while downregulating sphingolipids (3-ketosphingosine) and sugars (gentiobiose). These shifts suggest interference with membrane integrity and energy metabolism, providing a model for studying steroid-induced cytotoxicity .
Q. What experimental design considerations are critical for assessing this compound’s precision in pharmacokinetic studies?
Key parameters include:
- Concentration-dependent variability : At 1 pg/mg, clostebol exhibits higher CV% (20–30%) than its acetate (14–15%), necessitating replicate measurements at low doses .
- Matrix effects : Use deuterated analogs (e.g., clostebol-d3) as internal standards to correct for ionization suppression in biological matrices .
- Storage stability : Freeze-dried samples stored at −80°C show <10% degradation over six months, whereas room-temperature storage increases bias to −9% .
Properties
IUPAC Name |
[(8R,9S,10R,13S,14S,17S)-4-chloro-10,13-dimethyl-3-oxo-1,2,6,7,8,9,11,12,14,15,16,17-dodecahydrocyclopenta[a]phenanthren-17-yl] acetate | |
---|---|---|
Source | PubChem | |
URL | https://pubchem.ncbi.nlm.nih.gov | |
Description | Data deposited in or computed by PubChem | |
InChI |
InChI=1S/C21H29ClO3/c1-12(23)25-18-7-6-14-13-4-5-16-19(22)17(24)9-11-20(16,2)15(13)8-10-21(14,18)3/h13-15,18H,4-11H2,1-3H3/t13-,14-,15-,18-,20+,21-/m0/s1 | |
Source | PubChem | |
URL | https://pubchem.ncbi.nlm.nih.gov | |
Description | Data deposited in or computed by PubChem | |
InChI Key |
XYGMEFJSKQEBTO-KUJXMBTLSA-N | |
Source | PubChem | |
URL | https://pubchem.ncbi.nlm.nih.gov | |
Description | Data deposited in or computed by PubChem | |
Canonical SMILES |
CC(=O)OC1CCC2C1(CCC3C2CCC4=C(C(=O)CCC34C)Cl)C | |
Source | PubChem | |
URL | https://pubchem.ncbi.nlm.nih.gov | |
Description | Data deposited in or computed by PubChem | |
Isomeric SMILES |
CC(=O)O[C@H]1CC[C@@H]2[C@@]1(CC[C@H]3[C@H]2CCC4=C(C(=O)CC[C@]34C)Cl)C | |
Source | PubChem | |
URL | https://pubchem.ncbi.nlm.nih.gov | |
Description | Data deposited in or computed by PubChem | |
Molecular Formula |
C21H29ClO3 | |
Source | PubChem | |
URL | https://pubchem.ncbi.nlm.nih.gov | |
Description | Data deposited in or computed by PubChem | |
DSSTOX Substance ID |
DTXSID00234702 | |
Record name | Clostebol acetate | |
Source | EPA DSSTox | |
URL | https://comptox.epa.gov/dashboard/DTXSID00234702 | |
Description | DSSTox provides a high quality public chemistry resource for supporting improved predictive toxicology. | |
Molecular Weight |
364.9 g/mol | |
Source | PubChem | |
URL | https://pubchem.ncbi.nlm.nih.gov | |
Description | Data deposited in or computed by PubChem | |
CAS No. |
855-19-6 | |
Record name | (17β)-17-(Acetyloxy)-4-chloroandrost-4-en-3-one | |
Source | CAS Common Chemistry | |
URL | https://commonchemistry.cas.org/detail?cas_rn=855-19-6 | |
Description | CAS Common Chemistry is an open community resource for accessing chemical information. Nearly 500,000 chemical substances from CAS REGISTRY cover areas of community interest, including common and frequently regulated chemicals, and those relevant to high school and undergraduate chemistry classes. This chemical information, curated by our expert scientists, is provided in alignment with our mission as a division of the American Chemical Society. | |
Explanation | The data from CAS Common Chemistry is provided under a CC-BY-NC 4.0 license, unless otherwise stated. | |
Record name | Clostebol acetate | |
Source | ChemIDplus | |
URL | https://pubchem.ncbi.nlm.nih.gov/substance/?source=chemidplus&sourceid=0000855196 | |
Description | ChemIDplus is a free, web search system that provides access to the structure and nomenclature authority files used for the identification of chemical substances cited in National Library of Medicine (NLM) databases, including the TOXNET system. | |
Record name | Clostebol acetate | |
Source | DrugBank | |
URL | https://www.drugbank.ca/drugs/DB15984 | |
Description | The DrugBank database is a unique bioinformatics and cheminformatics resource that combines detailed drug (i.e. chemical, pharmacological and pharmaceutical) data with comprehensive drug target (i.e. sequence, structure, and pathway) information. | |
Explanation | Creative Common's Attribution-NonCommercial 4.0 International License (http://creativecommons.org/licenses/by-nc/4.0/legalcode) | |
Record name | Clostebol acetate | |
Source | DTP/NCI | |
URL | https://dtp.cancer.gov/dtpstandard/servlet/dwindex?searchtype=NSC&outputformat=html&searchlist=72159 | |
Description | The NCI Development Therapeutics Program (DTP) provides services and resources to the academic and private-sector research communities worldwide to facilitate the discovery and development of new cancer therapeutic agents. | |
Explanation | Unless otherwise indicated, all text within NCI products is free of copyright and may be reused without our permission. Credit the National Cancer Institute as the source. | |
Record name | Clostebol acetate | |
Source | EPA DSSTox | |
URL | https://comptox.epa.gov/dashboard/DTXSID00234702 | |
Description | DSSTox provides a high quality public chemistry resource for supporting improved predictive toxicology. | |
Record name | Clostebol acetate | |
Source | European Chemicals Agency (ECHA) | |
URL | https://echa.europa.eu/substance-information/-/substanceinfo/100.011.565 | |
Description | The European Chemicals Agency (ECHA) is an agency of the European Union which is the driving force among regulatory authorities in implementing the EU's groundbreaking chemicals legislation for the benefit of human health and the environment as well as for innovation and competitiveness. | |
Explanation | Use of the information, documents and data from the ECHA website is subject to the terms and conditions of this Legal Notice, and subject to other binding limitations provided for under applicable law, the information, documents and data made available on the ECHA website may be reproduced, distributed and/or used, totally or in part, for non-commercial purposes provided that ECHA is acknowledged as the source: "Source: European Chemicals Agency, http://echa.europa.eu/". Such acknowledgement must be included in each copy of the material. ECHA permits and encourages organisations and individuals to create links to the ECHA website under the following cumulative conditions: Links can only be made to webpages that provide a link to the Legal Notice page. | |
Record name | CLOSTEBOL ACETATE | |
Source | FDA Global Substance Registration System (GSRS) | |
URL | https://gsrs.ncats.nih.gov/ginas/app/beta/substances/780ZZX4P14 | |
Description | The FDA Global Substance Registration System (GSRS) enables the efficient and accurate exchange of information on what substances are in regulated products. Instead of relying on names, which vary across regulatory domains, countries, and regions, the GSRS knowledge base makes it possible for substances to be defined by standardized, scientific descriptions. | |
Explanation | Unless otherwise noted, the contents of the FDA website (www.fda.gov), both text and graphics, are not copyrighted. They are in the public domain and may be republished, reprinted and otherwise used freely by anyone without the need to obtain permission from FDA. Credit to the U.S. Food and Drug Administration as the source is appreciated but not required. | |
Retrosynthesis Analysis
AI-Powered Synthesis Planning: Our tool employs the Template_relevance Pistachio, Template_relevance Bkms_metabolic, Template_relevance Pistachio_ringbreaker, Template_relevance Reaxys, Template_relevance Reaxys_biocatalysis model, leveraging a vast database of chemical reactions to predict feasible synthetic routes.
One-Step Synthesis Focus: Specifically designed for one-step synthesis, it provides concise and direct routes for your target compounds, streamlining the synthesis process.
Accurate Predictions: Utilizing the extensive PISTACHIO, BKMS_METABOLIC, PISTACHIO_RINGBREAKER, REAXYS, REAXYS_BIOCATALYSIS database, our tool offers high-accuracy predictions, reflecting the latest in chemical research and data.
Strategy Settings
Precursor scoring | Relevance Heuristic |
---|---|
Min. plausibility | 0.01 |
Model | Template_relevance |
Template Set | Pistachio/Bkms_metabolic/Pistachio_ringbreaker/Reaxys/Reaxys_biocatalysis |
Top-N result to add to graph | 6 |
Feasible Synthetic Routes
Disclaimer and Information on In-Vitro Research Products
Please be aware that all articles and product information presented on BenchChem are intended solely for informational purposes. The products available for purchase on BenchChem are specifically designed for in-vitro studies, which are conducted outside of living organisms. In-vitro studies, derived from the Latin term "in glass," involve experiments performed in controlled laboratory settings using cells or tissues. It is important to note that these products are not categorized as medicines or drugs, and they have not received approval from the FDA for the prevention, treatment, or cure of any medical condition, ailment, or disease. We must emphasize that any form of bodily introduction of these products into humans or animals is strictly prohibited by law. It is essential to adhere to these guidelines to ensure compliance with legal and ethical standards in research and experimentation.