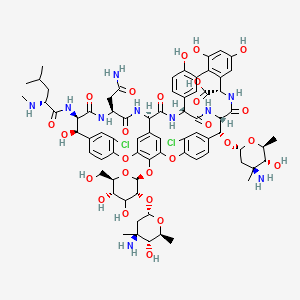
Chloroeremomycin
Overview
Description
Chloroeremomycin is a member of the glycopeptide family of antibiotics, similar to vancomycin. It is naturally produced by the bacterium Amycolatopsis orientalis. This compound is known for its ability to inhibit the construction of bacterial cell walls, making it a potent antibiotic. Although this compound itself has not been used in human medicine, its semi-synthetic derivative, oritavancin, has received FDA approval for treating bacterial infections .
Mechanism of Action
Target of Action
Chloroeremomycin, a member of the glycopeptide family of antibiotics, primarily targets the cell wall of Gram-positive bacteria . It binds to the D-alanyl-D-alanine stem termini in these bacteria, which are crucial for peptidoglycan synthesis .
Mode of Action
The compound inhibits transglycosylation, a key step in peptidoglycan synthesis, by binding to the D-alanyl-D-alanine stem termini . This interaction blocks the construction of the bacterial cell wall . Additionally, this compound also binds to the pentaglycyl bridging segment in peptidoglycan, distinguishing it from other glycopeptides . The presence of a hydrophobic group allows for interaction and disruption of the cell membrane, leading to depolarization, permeabilization, and rapid cell death .
Biochemical Pathways
This compound affects the biochemical pathway responsible for the synthesis of peptidoglycan, a critical component of the bacterial cell wall . By inhibiting transglycosylation and transpeptidation, it disrupts the formation of the peptidoglycan layer, thereby compromising the structural integrity of the bacterial cell wall .
Result of Action
The action of this compound results in the disruption of the bacterial cell wall and membrane, leading to cell death . Its activity is effective against both vancomycin-susceptible and -resistant organisms, as well as biofilm-producing Gram-positive bacteria .
Biochemical Analysis
Biochemical Properties
Chloroeremomycin plays a crucial role in biochemical reactions by inhibiting the synthesis of peptidoglycan, an essential component of bacterial cell walls. It interacts with enzymes such as transglycosylases and transpeptidases, which are involved in the cross-linking of peptidoglycan strands. By binding to the D-alanyl-D-alanine termini of peptidoglycan precursors, this compound prevents the proper formation of the cell wall, leading to bacterial cell death .
Cellular Effects
This compound affects various types of cells, particularly Gram-positive bacteria. It disrupts cell wall synthesis, leading to cell lysis and death. Additionally, this compound influences cell signaling pathways and gene expression by interfering with the normal function of cell wall synthesis enzymes. This disruption can lead to changes in cellular metabolism and overall cell function .
Molecular Mechanism
The molecular mechanism of this compound involves binding to the D-alanyl-D-alanine termini of peptidoglycan precursors, thereby inhibiting the action of transglycosylases and transpeptidases. This binding prevents the cross-linking of peptidoglycan strands, which is essential for maintaining the structural integrity of the bacterial cell wall. As a result, the bacterial cell becomes vulnerable to osmotic pressure and ultimately undergoes lysis .
Temporal Effects in Laboratory Settings
In laboratory settings, the effects of this compound have been observed to change over time. The compound is relatively stable, but its activity can degrade under certain conditions. Long-term studies have shown that this compound can maintain its antibacterial activity for extended periods, although some degradation may occur. The long-term effects on cellular function include sustained inhibition of cell wall synthesis and potential development of resistance in bacterial populations .
Dosage Effects in Animal Models
The effects of this compound vary with different dosages in animal models. At lower doses, the compound effectively inhibits bacterial growth without causing significant toxicity. At higher doses, this compound can exhibit toxic effects, including nephrotoxicity and ototoxicity. These adverse effects highlight the importance of determining the optimal dosage for therapeutic use .
Metabolic Pathways
This compound is involved in several metabolic pathways, including the biosynthesis of glycopeptide antibiotics. The compound undergoes various modifications, such as oxidative cross-linking of aromatic rings, hydroxylation, chlorination, methylation, and glycosylation. Enzymes such as OxyA-C catalyze these modifications, which are essential for the maturation and activity of this compound .
Transport and Distribution
Within cells and tissues, this compound is transported and distributed through interactions with specific transporters and binding proteins. These interactions facilitate the localization and accumulation of the compound in target bacterial cells, enhancing its antibacterial activity. The distribution of this compound within tissues is crucial for its effectiveness in treating infections .
Subcellular Localization
This compound primarily localizes to the bacterial cell wall, where it exerts its inhibitory effects on peptidoglycan synthesis. The compound’s targeting signals and post-translational modifications direct it to specific compartments within the bacterial cell, ensuring its effective action against the cell wall synthesis machinery .
Preparation Methods
Synthetic Routes and Reaction Conditions: Chloroeremomycin is produced through a complex biosynthetic pathway involving several enzymatic reactions. The genes responsible for its biosynthesis have been identified and sequenced, allowing for detailed studies on its production .
Industrial Production Methods: Industrial production of this compound involves fermentation using Amycolatopsis orientalis. The process includes optimizing the fermentation conditions to maximize yield and minimize impurities. Genetic engineering techniques, such as overexpressing specific genes, have been employed to enhance production efficiency .
Chemical Reactions Analysis
Types of Reactions: Chloroeremomycin undergoes various chemical reactions, including halogenation, glycosylation, methylation, oxidative cross-linking, and hydroxylation .
Common Reagents and Conditions:
Halogenation: Involves the addition of chlorine atoms to the molecule.
Glycosylation: Addition of sugar moieties to the peptide backbone.
Methylation: Introduction of methyl groups to specific sites on the molecule.
Oxidative Cross-Linking: Formation of covalent bonds between different parts of the molecule through oxidation.
Hydroxylation: Addition of hydroxyl groups to aromatic rings.
Major Products: The major products formed from these reactions include various derivatives of this compound, such as oritavancin, which has enhanced antibacterial properties .
Scientific Research Applications
Chloroeremomycin has several scientific research applications:
Chemistry: Used as a model compound to study glycopeptide antibiotics and their biosynthesis.
Biology: Investigated for its role in bacterial cell wall synthesis and resistance mechanisms.
Comparison with Similar Compounds
Chloroeremomycin is similar to other glycopeptide antibiotics such as vancomycin and teicoplanin. it has unique features that distinguish it from these compounds:
Properties
IUPAC Name |
(1S,2R,18R,19R,22S,25R,28R,40S)-2-[(2R,4S,5R,6S)-4-amino-5-hydroxy-4,6-dimethyloxan-2-yl]oxy-48-[(2S,3R,4S,5S,6R)-3-[(2R,4S,5R,6S)-4-amino-5-hydroxy-4,6-dimethyloxan-2-yl]oxy-4,5-dihydroxy-6-(hydroxymethyl)oxan-2-yl]oxy-22-(2-amino-2-oxoethyl)-5,15-dichloro-18,32,35,37-tetrahydroxy-19-[[(2R)-4-methyl-2-(methylamino)pentanoyl]amino]-20,23,26,42,44-pentaoxo-7,13-dioxa-21,24,27,41,43-pentazaoctacyclo[26.14.2.23,6.214,17.18,12.129,33.010,25.034,39]pentaconta-3,5,8,10,12(48),14,16,29(45),30,32,34(39),35,37,46,49-pentadecaene-40-carboxylic acid | |
---|---|---|
Source | PubChem | |
URL | https://pubchem.ncbi.nlm.nih.gov | |
Description | Data deposited in or computed by PubChem | |
InChI |
InChI=1S/C73H88Cl2N10O26/c1-26(2)14-38(79-7)64(96)84-54-56(91)30-9-12-42(36(74)16-30)106-44-18-32-19-45(60(44)111-71-61(58(93)57(92)46(25-86)108-71)110-49-24-73(6,78)63(95)28(4)105-49)107-43-13-10-31(17-37(43)75)59(109-48-23-72(5,77)62(94)27(3)104-48)55-69(101)83-53(70(102)103)35-20-33(87)21-41(89)50(35)34-15-29(8-11-40(34)88)51(66(98)85-55)82-67(99)52(32)81-65(97)39(22-47(76)90)80-68(54)100/h8-13,15-21,26-28,38-39,46,48-49,51-59,61-63,71,79,86-89,91-95H,14,22-25,77-78H2,1-7H3,(H2,76,90)(H,80,100)(H,81,97)(H,82,99)(H,83,101)(H,84,96)(H,85,98)(H,102,103)/t27-,28-,38+,39-,46+,48-,49-,51+,52+,53-,54+,55-,56+,57+,58-,59+,61+,62-,63-,71-,72-,73-/m0/s1 | |
Source | PubChem | |
URL | https://pubchem.ncbi.nlm.nih.gov | |
Description | Data deposited in or computed by PubChem | |
InChI Key |
XJHXLMVKYIVZTE-LOALFDMRSA-N | |
Source | PubChem | |
URL | https://pubchem.ncbi.nlm.nih.gov | |
Description | Data deposited in or computed by PubChem | |
Canonical SMILES |
CC1C(C(CC(O1)OC2C(C(C(OC2OC3=C4C=C5C=C3OC6=C(C=C(C=C6)C(C7C(=O)NC(C8=C(C(=CC(=C8)O)O)C9=C(C=CC(=C9)C(C(=O)N7)NC(=O)C5NC(=O)C(NC(=O)C(C(C1=CC(=C(O4)C=C1)Cl)O)NC(=O)C(CC(C)C)NC)CC(=O)N)O)C(=O)O)OC1CC(C(C(O1)C)O)(C)N)Cl)CO)O)O)(C)N)O | |
Source | PubChem | |
URL | https://pubchem.ncbi.nlm.nih.gov | |
Description | Data deposited in or computed by PubChem | |
Isomeric SMILES |
C[C@H]1[C@@H]([C@@](C[C@@H](O1)O[C@@H]2[C@H]([C@@H]([C@H](O[C@H]2OC3=C4C=C5C=C3OC6=C(C=C(C=C6)[C@H]([C@H]7C(=O)N[C@@H](C8=C(C(=CC(=C8)O)O)C9=C(C=CC(=C9)[C@H](C(=O)N7)NC(=O)[C@@H]5NC(=O)[C@@H](NC(=O)[C@@H]([C@@H](C1=CC(=C(O4)C=C1)Cl)O)NC(=O)[C@@H](CC(C)C)NC)CC(=O)N)O)C(=O)O)O[C@H]1C[C@]([C@H]([C@@H](O1)C)O)(C)N)Cl)CO)O)O)(C)N)O | |
Source | PubChem | |
URL | https://pubchem.ncbi.nlm.nih.gov | |
Description | Data deposited in or computed by PubChem | |
Molecular Formula |
C73H88Cl2N10O26 | |
Source | PubChem | |
URL | https://pubchem.ncbi.nlm.nih.gov | |
Description | Data deposited in or computed by PubChem | |
Molecular Weight |
1592.4 g/mol | |
Source | PubChem | |
URL | https://pubchem.ncbi.nlm.nih.gov | |
Description | Data deposited in or computed by PubChem | |
CAS No. |
118395-73-6 | |
Record name | Chloroeremomycin | |
Source | CAS Common Chemistry | |
URL | https://commonchemistry.cas.org/detail?cas_rn=118395-73-6 | |
Description | CAS Common Chemistry is an open community resource for accessing chemical information. Nearly 500,000 chemical substances from CAS REGISTRY cover areas of community interest, including common and frequently regulated chemicals, and those relevant to high school and undergraduate chemistry classes. This chemical information, curated by our expert scientists, is provided in alignment with our mission as a division of the American Chemical Society. | |
Explanation | The data from CAS Common Chemistry is provided under a CC-BY-NC 4.0 license, unless otherwise stated. | |
Record name | CHLOROEREMOMYCIN | |
Source | FDA Global Substance Registration System (GSRS) | |
URL | https://gsrs.ncats.nih.gov/ginas/app/beta/substances/X47SLZ2K7D | |
Description | The FDA Global Substance Registration System (GSRS) enables the efficient and accurate exchange of information on what substances are in regulated products. Instead of relying on names, which vary across regulatory domains, countries, and regions, the GSRS knowledge base makes it possible for substances to be defined by standardized, scientific descriptions. | |
Explanation | Unless otherwise noted, the contents of the FDA website (www.fda.gov), both text and graphics, are not copyrighted. They are in the public domain and may be republished, reprinted and otherwise used freely by anyone without the need to obtain permission from FDA. Credit to the U.S. Food and Drug Administration as the source is appreciated but not required. | |
Retrosynthesis Analysis
AI-Powered Synthesis Planning: Our tool employs the Template_relevance Pistachio, Template_relevance Bkms_metabolic, Template_relevance Pistachio_ringbreaker, Template_relevance Reaxys, Template_relevance Reaxys_biocatalysis model, leveraging a vast database of chemical reactions to predict feasible synthetic routes.
One-Step Synthesis Focus: Specifically designed for one-step synthesis, it provides concise and direct routes for your target compounds, streamlining the synthesis process.
Accurate Predictions: Utilizing the extensive PISTACHIO, BKMS_METABOLIC, PISTACHIO_RINGBREAKER, REAXYS, REAXYS_BIOCATALYSIS database, our tool offers high-accuracy predictions, reflecting the latest in chemical research and data.
Strategy Settings
Precursor scoring | Relevance Heuristic |
---|---|
Min. plausibility | 0.01 |
Model | Template_relevance |
Template Set | Pistachio/Bkms_metabolic/Pistachio_ringbreaker/Reaxys/Reaxys_biocatalysis |
Top-N result to add to graph | 6 |
Feasible Synthetic Routes
A: Chloroeremomycin, a member of the vancomycin family of glycopeptide antibiotics, exerts its antibacterial activity by binding to the D-Ala-D-Ala terminus of peptidoglycan precursors on the bacterial cell wall. [, , ] This binding inhibits both transglycosylation and transpeptidation reactions, crucial steps in bacterial cell wall biosynthesis. [, ] The disruption of these processes leads to weakened cell wall integrity, ultimately causing bacterial cell death. []
A: While the provided abstracts don't explicitly detail the molecular formula and spectroscopic data of this compound, they highlight its structural similarities to vancomycin and mention key features like the presence of three sugars: one D-glucose and two L-4-epi-vancosamines. [, ] These sugars are attached to a crosslinked heptapeptide backbone. [] Determining the exact molecular formula and weight requires accessing the full scientific literature on this compound. Spectroscopic data like NMR and mass spectrometry details, vital for structural elucidation, would also be found in comprehensive research articles rather than abstracts. [, ]
A: The provided abstracts primarily focus on the biochemical mechanisms and structure-activity relationships of this compound. [, , , ] Information regarding its material compatibility and stability under various conditions is not discussed in these specific research snippets. Exploring the broader scientific literature on this compound, particularly studies focused on its formulation and storage, would be necessary to answer this question comprehensively.
A: Yes, computational chemistry techniques have been employed to investigate the binding interactions and cooperative behavior of this compound. [] Molecular dynamics simulations and quasiharmonic normal-mode analysis were used to study the configurational entropy changes associated with ligand binding and dimerization of this compound. [] These studies provide insights into the thermodynamic driving forces contributing to the enhanced activity of this compound compared to other glycopeptide antibiotics. [] Further computational studies focusing on QSAR model development would be valuable for predicting the activity of novel this compound derivatives.
A: Modifications to the this compound structure, particularly at the vancosamine sugar, significantly impact its activity. [, , ] For example, adding a 4′-chlorobiphenylmethyl group to the vancosamine moiety, as seen in oritavancin (a semi-synthetic derivative of this compound), enhances its activity against vancomycin-resistant enterococci (VRE) and Staphylococcus aureus (VRSA). [, , ] This modification is believed to provide additional interactions with the bacterial cell wall and enhance the drug's ability to inhibit transglycosylation. [, , ] Other modifications, such as those impacting the D-Ala-D-Ala binding site, can affect the drug's ability to inhibit transpeptidation. [] Understanding the SAR of this compound is crucial for developing new derivatives with improved potency and efficacy against resistant bacterial strains.
A: While the provided abstracts don't explicitly address the stability and formulation of this compound, they highlight the importance of its sugar moieties for its activity. [, , , ] The stability of these sugar moieties under various conditions (e.g., pH, temperature) would be a crucial factor affecting the overall stability of the drug. Moreover, as a glycopeptide antibiotic, this compound's solubility and bioavailability might present challenges. Further research would be required to understand the impact of different formulation strategies (e.g., use of excipients, encapsulation techniques) on its stability, solubility, and bioavailability.
Disclaimer and Information on In-Vitro Research Products
Please be aware that all articles and product information presented on BenchChem are intended solely for informational purposes. The products available for purchase on BenchChem are specifically designed for in-vitro studies, which are conducted outside of living organisms. In-vitro studies, derived from the Latin term "in glass," involve experiments performed in controlled laboratory settings using cells or tissues. It is important to note that these products are not categorized as medicines or drugs, and they have not received approval from the FDA for the prevention, treatment, or cure of any medical condition, ailment, or disease. We must emphasize that any form of bodily introduction of these products into humans or animals is strictly prohibited by law. It is essential to adhere to these guidelines to ensure compliance with legal and ethical standards in research and experimentation.