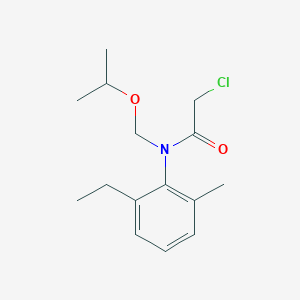
Propisochlor
Overview
Description
Propisochlor is a chloroacetamide herbicide widely used in agriculture to control annual grasses and some broad-leaved weeds. It is primarily applied as a pre-emergence or pre-plant herbicide in crops such as maize, potatoes, soybeans, corn, peanuts, rice, and wheat . The compound is known for its selective action, being absorbed by the shoots of germinating plants and inhibiting protein synthesis, which blocks cell division .
Mechanism of Action
Target of Action
Propisochlor, a chloroacetanilide herbicide, primarily targets the protein synthesis machinery in plants . It inhibits protein synthesis, thereby blocking cell division .
Mode of Action
This compound acts selectively, being absorbed by the shoots of germinating plants . It inhibits the synthesis of very long-chain fatty acids (VLCFA), which are essential for cell division . This inhibition disrupts the normal growth and development of the plant, leading to its eventual death .
Biochemical Pathways
The primary biochemical pathway affected by this compound is the protein synthesis pathway . By inhibiting protein synthesis, this compound disrupts the normal functioning of the plant cells, leading to impaired growth and development . A study has shown that a bifunctional enzyme belonging to the cytochrome P450 family is involved in the O-dealkylation and N-dealkoxymethylation of this compound .
Pharmacokinetics
It is known that this compound is moderately mobile in the environment
Result of Action
The molecular and cellular effects of this compound’s action include impaired gut morphology, reduced expression of tight junction proteins, decreased thickness of the mucus layer, and activation of pyroptosis signaling . These effects result from the compound’s inhibition of protein synthesis and cell division .
Action Environment
Environmental factors can influence the action, efficacy, and stability of this compound. For example, its mobility in the environment can affect its distribution and hence its efficacy . Additionally, the presence of certain bacteria in the environment can influence the degradation of this compound . .
Biochemical Analysis
Biochemical Properties
Propisochlor interacts with various enzymes and proteins in the plant cells. It inhibits the synthesis of protein, thereby preventing the growth of the target weeds .
Cellular Effects
This compound affects various types of cells and cellular processes. It influences cell function by suppressing protein synthesis, which in turn affects cell signaling pathways, gene expression, and cellular metabolism .
Molecular Mechanism
The molecular mechanism of action of this compound involves binding interactions with biomolecules, enzyme inhibition, and changes in gene expression. By inhibiting protein synthesis, this compound disrupts the normal functioning of the cell .
Temporal Effects in Laboratory Settings
In laboratory settings, the effects of this compound change over time. The half-life times (t1/2) in soil was 5.541 and 6.074 days, respectively . No this compound residues (<0.01 mg/kg) were detected in corn at harvest time withholding period of 2.5 months after treatments of the pesticides .
Preparation Methods
Synthetic Routes and Reaction Conditions: Propisochlor is synthesized through a series of chemical reactions involving the chlorination of acetanilide derivatives. The process typically involves the reaction of 2-chloro-N-isopropylacetanilide with ethyl chloroformate in the presence of a base such as sodium hydroxide. The reaction conditions include maintaining a temperature range of 0-5°C to ensure the selective formation of the desired product.
Industrial Production Methods: In industrial settings, the production of this compound involves large-scale chlorination processes. The raw materials are mixed in reactors equipped with temperature control systems to maintain the optimal reaction conditions. The product is then purified through distillation and crystallization processes to achieve the required purity levels for agricultural use .
Chemical Reactions Analysis
Types of Reactions: Propisochlor undergoes various chemical reactions, including:
Oxidation: this compound can be oxidized to form corresponding sulfoxides and sulfones.
Reduction: The compound can be reduced to form amines.
Substitution: this compound can undergo nucleophilic substitution reactions, where the chlorine atom is replaced by other nucleophiles.
Common Reagents and Conditions:
Oxidation: Common oxidizing agents include potassium permanganate and hydrogen peroxide.
Reduction: Reducing agents such as lithium aluminum hydride and sodium borohydride are used.
Substitution: Nucleophiles like hydroxide ions and amines are commonly used in substitution reactions.
Major Products Formed:
Oxidation: Sulfoxides and sulfones.
Reduction: Amines.
Substitution: Various substituted derivatives depending on the nucleophile used.
Scientific Research Applications
Propisochlor has several scientific research applications, including:
Chemistry: Used as a model compound in studying the behavior of chloroacetamide herbicides in various chemical reactions.
Biology: Investigated for its effects on plant physiology and its role in inhibiting protein synthesis.
Comparison with Similar Compounds
Propisochlor belongs to the chloroacetamide family of herbicides, which includes similar compounds such as acetochlor, metolachlor, and alachlor. Compared to these compounds, this compound is unique in its specific molecular structure, which provides a distinct mode of action and selectivity. For instance:
Acetochlor: Similar in structure but differs in the alkyl side chain, leading to variations in herbicidal activity.
Metolachlor: Has a different stereochemistry, affecting its absorption and efficacy.
Alachlor: Similar mode of action but differs in its environmental persistence and toxicity profile.
This compound’s unique structure and selective action make it a valuable herbicide in agricultural practices, offering effective weed control with specific applications in various crops.
Properties
IUPAC Name |
2-chloro-N-(2-ethyl-6-methylphenyl)-N-(propan-2-yloxymethyl)acetamide | |
---|---|---|
Source | PubChem | |
URL | https://pubchem.ncbi.nlm.nih.gov | |
Description | Data deposited in or computed by PubChem | |
InChI |
InChI=1S/C15H22ClNO2/c1-5-13-8-6-7-12(4)15(13)17(14(18)9-16)10-19-11(2)3/h6-8,11H,5,9-10H2,1-4H3 | |
Source | PubChem | |
URL | https://pubchem.ncbi.nlm.nih.gov | |
Description | Data deposited in or computed by PubChem | |
InChI Key |
KZNDFYDURHAESM-UHFFFAOYSA-N | |
Source | PubChem | |
URL | https://pubchem.ncbi.nlm.nih.gov | |
Description | Data deposited in or computed by PubChem | |
Canonical SMILES |
CCC1=CC=CC(=C1N(COC(C)C)C(=O)CCl)C | |
Source | PubChem | |
URL | https://pubchem.ncbi.nlm.nih.gov | |
Description | Data deposited in or computed by PubChem | |
Molecular Formula |
C15H22ClNO2 | |
Source | PubChem | |
URL | https://pubchem.ncbi.nlm.nih.gov | |
Description | Data deposited in or computed by PubChem | |
DSSTOX Substance ID |
DTXSID2058205 | |
Record name | Propisochlor | |
Source | EPA DSSTox | |
URL | https://comptox.epa.gov/dashboard/DTXSID2058205 | |
Description | DSSTox provides a high quality public chemistry resource for supporting improved predictive toxicology. | |
Molecular Weight |
283.79 g/mol | |
Source | PubChem | |
URL | https://pubchem.ncbi.nlm.nih.gov | |
Description | Data deposited in or computed by PubChem | |
CAS No. |
86763-47-5 | |
Record name | Propisochlor | |
Source | CAS Common Chemistry | |
URL | https://commonchemistry.cas.org/detail?cas_rn=86763-47-5 | |
Description | CAS Common Chemistry is an open community resource for accessing chemical information. Nearly 500,000 chemical substances from CAS REGISTRY cover areas of community interest, including common and frequently regulated chemicals, and those relevant to high school and undergraduate chemistry classes. This chemical information, curated by our expert scientists, is provided in alignment with our mission as a division of the American Chemical Society. | |
Explanation | The data from CAS Common Chemistry is provided under a CC-BY-NC 4.0 license, unless otherwise stated. | |
Record name | Propisochlor [ISO] | |
Source | ChemIDplus | |
URL | https://pubchem.ncbi.nlm.nih.gov/substance/?source=chemidplus&sourceid=0086763475 | |
Description | ChemIDplus is a free, web search system that provides access to the structure and nomenclature authority files used for the identification of chemical substances cited in National Library of Medicine (NLM) databases, including the TOXNET system. | |
Record name | Propisochlor | |
Source | EPA DSSTox | |
URL | https://comptox.epa.gov/dashboard/DTXSID2058205 | |
Description | DSSTox provides a high quality public chemistry resource for supporting improved predictive toxicology. | |
Record name | PROPISOCHLOR | |
Source | FDA Global Substance Registration System (GSRS) | |
URL | https://gsrs.ncats.nih.gov/ginas/app/beta/substances/88248R3YQC | |
Description | The FDA Global Substance Registration System (GSRS) enables the efficient and accurate exchange of information on what substances are in regulated products. Instead of relying on names, which vary across regulatory domains, countries, and regions, the GSRS knowledge base makes it possible for substances to be defined by standardized, scientific descriptions. | |
Explanation | Unless otherwise noted, the contents of the FDA website (www.fda.gov), both text and graphics, are not copyrighted. They are in the public domain and may be republished, reprinted and otherwise used freely by anyone without the need to obtain permission from FDA. Credit to the U.S. Food and Drug Administration as the source is appreciated but not required. | |
Retrosynthesis Analysis
AI-Powered Synthesis Planning: Our tool employs the Template_relevance Pistachio, Template_relevance Bkms_metabolic, Template_relevance Pistachio_ringbreaker, Template_relevance Reaxys, Template_relevance Reaxys_biocatalysis model, leveraging a vast database of chemical reactions to predict feasible synthetic routes.
One-Step Synthesis Focus: Specifically designed for one-step synthesis, it provides concise and direct routes for your target compounds, streamlining the synthesis process.
Accurate Predictions: Utilizing the extensive PISTACHIO, BKMS_METABOLIC, PISTACHIO_RINGBREAKER, REAXYS, REAXYS_BIOCATALYSIS database, our tool offers high-accuracy predictions, reflecting the latest in chemical research and data.
Strategy Settings
Precursor scoring | Relevance Heuristic |
---|---|
Min. plausibility | 0.01 |
Model | Template_relevance |
Template Set | Pistachio/Bkms_metabolic/Pistachio_ringbreaker/Reaxys/Reaxys_biocatalysis |
Top-N result to add to graph | 6 |
Feasible Synthetic Routes
Q1: What is the primary mechanism of action of Propisochlor?
A1: this compound inhibits the elongation of fatty acid chains, specifically targeting the enzyme Acetyl-CoA Carboxylase (ACCase), which is crucial for lipid biosynthesis in plants. This disruption of lipid production ultimately leads to the death of susceptible weeds.
Q2: How does the inhibition of fatty acid elongation affect weed growth?
A2: Fatty acids are essential building blocks for cell membranes, which are crucial for maintaining cell integrity and function. Disrupting fatty acid synthesis disrupts the formation of new cell membranes, ultimately leading to growth arrest and death in weeds.
Q3: What is the molecular formula and weight of this compound?
A3: this compound has the molecular formula C15H22ClNO2 and a molecular weight of 283.79 g/mol.
Q4: Is there any spectroscopic data available for this compound?
A4: Yes, several studies have characterized this compound using techniques like Nuclear Magnetic Resonance (NMR) spectroscopy, Gas Chromatography-Mass Spectrometry (GC-MS), and High Performance Liquid Chromatography (HPLC). These techniques provide insights into the compound's structure, purity, and degradation products. [], [], []
Q5: How stable is this compound under different environmental conditions?
A5: this compound exhibits varying stability depending on factors like temperature, pH, and the presence of light or other chemicals. Research shows that it degrades relatively quickly in water, with half-lives ranging from 1.0 to 5.7 days depending on the location. [] In soil, its persistence is longer, with reported half-lives ranging from 4.7 to 21.2 days. [], [] Factors like soil organic matter content and temperature can significantly influence its degradation rate. [], [], []
Q6: How does the presence of organic matter in soil affect this compound persistence?
A6: Higher organic matter content generally leads to increased adsorption of this compound, reducing its mobility and potentially prolonging its persistence in the soil. This retention can influence its bioavailability to target weeds and its potential for leaching into groundwater. []
Q7: Does this compound exhibit any catalytic properties itself?
A7: this compound primarily acts as an inhibitor rather than a catalyst. Its mode of action involves binding to the ACCase enzyme, preventing it from catalyzing fatty acid elongation.
Q8: Have there been any computational studies on this compound?
A8: While limited information is available on computational studies specifically focused on this compound, techniques like Quantitative Structure-Activity Relationship (QSAR) modeling can be employed to predict its behavior and potential environmental fate based on its structure and physicochemical properties.
Q9: How do structural modifications of this compound affect its herbicidal activity?
A9: Structural modifications, particularly to the N-alkoxyalkyl substituent, can significantly influence the enantiomeric separation and potentially the biological activity of this compound. [], [] Understanding these relationships is crucial for designing new herbicides with improved efficacy and selectivity.
Q10: What are some formulation strategies for improving the stability or efficacy of this compound?
A10: Researchers have explored microencapsulation of this compound using poly(melamine-formaldehyde) to create a controlled-release formulation. This technology aims to enhance the herbicide's stability, prolong its activity, and potentially reduce its environmental impact by controlling its release. []
Q11: What are the recommended application rates for this compound in different crops?
A11: Recommended application rates vary depending on the crop, soil type, and target weeds. It is essential to consult the product label and local regulations for specific guidelines. Research suggests that a dosage of 2,100 mL/ha of 72% this compound EC, applied once, might be suitable for soybean fields, with a maximum residue limit (MRL) of 0.1 mg/kg in soybean suggested based on two-year field trials. []
Q12: What analytical methods are commonly used for detecting and quantifying this compound residues?
A12: Various techniques are employed for this compound analysis, including:
- Gas Chromatography with Electron Capture Detector (GC-ECD): This method is widely used for its sensitivity and selectivity in detecting this compound residues in soil, water, and plant samples. [], [], [], [], []
- High Performance Liquid Chromatography (HPLC): HPLC, often coupled with UV detection, offers a versatile approach for separating and quantifying this compound and its potential degradation products in various matrices. [], [], [], []
- Gas Chromatography-Mass Spectrometry (GC-MS): This technique provides structural information about this compound and its degradation products, aiding in understanding its environmental fate. [], []
- Fluorescence Polarization Immunoassay (FPIA): This rapid and sensitive method allows for on-site screening of this compound contamination in water samples. []
Q13: How does this compound behave in the environment, and what are its potential ecotoxicological effects?
A13: this compound primarily enters the environment through agricultural applications and can impact soil, water, and air quality.
- Soil: Its persistence in soil depends on various factors, including organic matter content, temperature, and microbial activity. This compound can potentially leach into groundwater, posing a risk to aquatic ecosystems. [], []
- Water: this compound is relatively mobile in water and can contaminate surface water bodies through runoff. Its degradation in water is influenced by factors like pH, temperature, and the presence of light and dissolved oxygen. [], [], [], []
Q14: What strategies can mitigate the negative environmental impacts of this compound?
A14: Several approaches can help minimize the ecological footprint of this compound:
- Bioremediation: Utilizing microorganisms capable of degrading this compound, like the Rhodococcus sp. B2 strain, shows promise for bioremediation of contaminated sites. This bacterium can break down this compound through both O-dealkylation and N-dealkoxymethylation processes. []
Q15: Are there any viable alternatives to this compound for weed control?
A15: Several alternative herbicides, including Acetochlor, Metolachlor, and Atrazine, are available, each with its own efficacy and selectivity profiles. Integrated weed management strategies often combine different herbicides with non-chemical control methods for more sustainable and effective weed control. [], [], [], []
Disclaimer and Information on In-Vitro Research Products
Please be aware that all articles and product information presented on BenchChem are intended solely for informational purposes. The products available for purchase on BenchChem are specifically designed for in-vitro studies, which are conducted outside of living organisms. In-vitro studies, derived from the Latin term "in glass," involve experiments performed in controlled laboratory settings using cells or tissues. It is important to note that these products are not categorized as medicines or drugs, and they have not received approval from the FDA for the prevention, treatment, or cure of any medical condition, ailment, or disease. We must emphasize that any form of bodily introduction of these products into humans or animals is strictly prohibited by law. It is essential to adhere to these guidelines to ensure compliance with legal and ethical standards in research and experimentation.