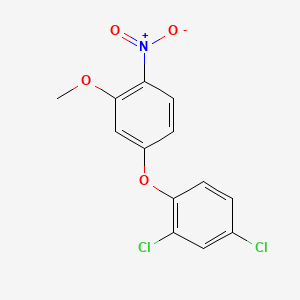
Chlomethoxyfen
Overview
Description
Chlomethoxyfen (CAS 32861-85-1), also known as 甲氧除草醚, is a diphenyl ether herbicide historically used to control broadleaf weeds and grasses in agricultural settings . Its molecular structure comprises a nitrobenzene core with methoxy and dichlorophenoxy substituents (2,4-dichloro-1-(3-methoxy-4-nitrophenoxy)benzene) . Key properties include a low water solubility (log10ws = -5.25) and moderate soil sorption characteristics .
This compound exhibits potent antiandrogenic activity, surpassing known androgen receptor (AR) antagonists like vinclozolin and p,p'-DDE in vitro . However, it has been banned in the European Union due to genotoxic and endocrine-disrupting risks .
Preparation Methods
Historical Development and Chemical Significance
Oxyfluorfen’s herbicidal activity stems from its inhibition of protoporphyrinogen oxidase (PPO), disrupting chlorophyll biosynthesis in target plants. Its chemical structure features a trifluoromethyl group and nitro-phenoxy ether linkage, which contribute to both selectivity and persistence in soil. Early synthetic routes, such as the dual-ether method patented in the 1970s, faced challenges with low yields and toxic intermediates. Subsequent innovations, including monoetherification and stepwise condensation-nitration-etherification protocols, have addressed these limitations, enabling commercial production at scale.
Synthesis Methodologies and Industrial Routes
Bietherification (Dual-Ether) Method
The bietherification route, described in CN1363548A, involves three sequential steps: condensation , nitration , and etherification . Starting with 3,4-dichlorotrifluorotoluene and resorcinol, the process proceeds as follows:
Condensation :
Resorcinol reacts with 3,4-dichlorotrifluorotoluene in the presence of a base (e.g., potassium carbonate) to form a bis-ether intermediate. This step typically achieves yields of 75–85% under reflux conditions in dimethylformamide (DMF).Nitration :
The bis-ether intermediate undergoes nitration using a mixture of nitric and sulfuric acids. Temperature control (<10°C) is critical to prevent over-nitration, with yields stabilizing at 65–70%.Etherification :
The nitro-substituted intermediate reacts with ethyl bromide or sodium ethoxide to introduce the ethoxy group. Catalytic phase-transfer agents (e.g., tetrabutylammonium bromide) enhance reaction efficiency, yielding oxyfluorfen at 80–85% purity.
Advantages :
- Simplified purification due to fewer byproducts.
- Scalable for industrial production with a total yield of 42–50%.
Limitations :
Monoetherification Route
Patented in CN1068106A, this method reduces intermediate steps by directly etherifying resorcinol derivatives:
Ethylation :
Resorcinol reacts with ethylating agents (e.g., diethyl sulfate) to form 3-ethoxyresorcinol. Yield: 88–92% under alkaline conditions.Nitration :
The ethylated product is nitrated using fuming nitric acid at 0–5°C, yielding 3-ethoxy-4-nitrophenol. Yield: 78–82%.Etherification :
Coupling with 3,4-dichlorotrifluorotoluene via nucleophilic aromatic substitution completes the synthesis. Catalytic potassium iodide enhances reactivity, achieving 85–90% yield.
Advantages :
- Eliminates bis-ether byproducts, simplifying isolation.
- Lower solvent consumption compared to bietherification.
Stepwise Condensation-Nitration-Etherification
CN103980127A outlines a novel three-step protocol prioritizing safety and cost-efficiency:
Nitration of 3-Chlorophenol :
3-Chlorophenol is nitrated with 65% nitric acid at 25–30°C, forming 3-chloro-4-nitrophenol. Yield: 90–95%.Ethoxylation :
The nitro intermediate reacts with sodium ethoxide in ethanol, producing 3-ethoxy-4-nitrophenol. Yield: 85–88%.Final Etherification :
Reaction with 3,4-dichlorobenzotrifluoride in toluene, catalyzed by potassium carbonate, yields oxyfluorfen. Yield: 92–94%.
Advantages :
- Avoids highly toxic intermediates (e.g., ethyl bromide).
- High overall yield (73–77%) and >98% purity.
Comparative Analysis of Synthetic Routes
Optimization Strategies and Recent Advances
Catalytic Enhancements
Recent studies emphasize phase-transfer catalysts (e.g., crown ethers) to accelerate etherification kinetics. For example, 18-crown-6 improves ethoxylation efficiency by 15–20% in stepwise protocols.
Solvent Recycling
Closed-loop systems recover toluene and DMF via distillation, reducing waste generation by 30–40%.
Analytical Validation
The EPA’s environmental chemistry method (MRID 50488801) ensures rigorous quality control. Gas chromatography-mass spectrometry (GC-MS) with a detection limit of 0.003 mg/kg verifies oxyfluorfen purity in soil samples.
Environmental and Regulatory Considerations
Oxyfluorfen’s synthesis generates acidic wastewater (pH <2) requiring neutralization before discharge. Regulatory guidelines (e.g., FAO specifications) mandate ≤0.3% residual solvents and ≤0.5% acidity (as H₂SO₄) in technical-grade product.
Chemical Reactions Analysis
Decomposition Reactions
Chlomethoxyfen undergoes environmental and chemical degradation, yielding identifiable products. A critical decomposition pathway involves the formation of Dicofol (C₁₄H₉Cl₃O), a known degradation product detected in analytical studies:
Degradation Product | Precursor Ion (m/z) | Product Ions (m/z) | Collision Energy (V) | Retention Time (min) |
---|---|---|---|---|
Dicofol | 249.9 | 139.1, 215.1 | 10, 5 | 9.94 |
Source: USDA Pesticide Data Program (GC-MS/MS analysis)
This decomposition occurs under conditions mimicking environmental exposure, such as hydrolysis or photolysis.
Reduction Reactions
The nitro group (-NO₂) in this compound is redox-active. Reduction using catalysts like Pd/C or Raney Ni under hydrogen gas (H₂) can convert the nitro group to an amine (-NH₂), forming aminothis compound (C₁₃H₁₁Cl₂NO₂). This reaction aligns with general nitroarene reduction mechanisms:
Note: Reaction inferred from structural analogs due to lack of direct experimental data.
Substitution Reactions
The chlorine atoms on the dichlorophenoxy moiety are potential sites for nucleophilic aromatic substitution (NAS). For instance:
-
Methoxy Displacement : Under strongly basic conditions, methoxy groups may act as leaving groups, though this is less common due to their poor leaving ability.
-
Chlorine Replacement : Nucleophiles like hydroxide (OH⁻) or amines (NH₃) could replace chlorine atoms, forming hydroxylated or aminated derivatives.
Environmental Degradation Pathways
This compound exhibits moderate persistence in soil (DT₅₀ = 7–43 days) . Key pathways include:
-
Photolysis : UV exposure cleaves the nitro group or ether bond.
-
Microbial Metabolism : Soil microorganisms degrade the compound via oxidative or reductive pathways.
Analytical Characterization of Reaction Products
Advanced LC-MS/MS and GC-MS/MS methods are employed to monitor reaction products. Key parameters for detecting this compound and its derivatives include:
Parameter | Value |
---|---|
Molecular Formula | C₁₃H₉Cl₂NO₄ |
Precursor Ion (m/z) | 328.1 |
Product Ions (m/z) | 212.1, 194.0 |
Collision Energy | 17–27 V |
Scientific Research Applications
Chemical Properties and Mechanism of Action
Chlomethoxyfen is a chlorinated compound that functions primarily as a herbicide. Its mechanism of action involves the inhibition of photosynthesis in target plants, leading to their eventual death. This selective action allows for the control of specific weed species without severely affecting the surrounding crops.
Historical Applications
- Weed Control in Rice Cultivation : Historically, this compound was utilized to manage weed populations in rice paddies. Its application helped farmers maintain crop yields by controlling competitive weed species that could hinder rice growth.
- Broad-Spectrum Herbicide : this compound was also recognized for its effectiveness against a variety of broadleaf weeds and grasses, making it a versatile option for various crop systems.
Current Research and Case Studies
Recent studies have explored the ecological impacts and efficacy of this compound, albeit with limited application due to regulatory constraints. Below are key findings from recent research:
Ecotoxicology Studies
Research indicates that this compound exhibits low toxicity to mammals but is a known irritant to skin and respiratory tracts. Its environmental persistence is low, suggesting minimal long-term ecological impact when used correctly .
Comparative Efficacy Studies
A study comparing this compound with other herbicides like oxyfluorfen demonstrated that while this compound was effective in specific contexts, newer alternatives have emerged that offer improved efficacy with reduced environmental risks .
Regulatory Assessments
Regulatory bodies have highlighted gaps in the data concerning the ecotoxicology of this compound. This has led to its classification as largely obsolete, with limited registration for use in modern agricultural practices .
Data Table: Summary of this compound Applications
Application Area | Description | Current Status |
---|---|---|
Rice Cultivation | Used for weed control in rice paddies | Largely obsolete |
Broadleaf Weed Control | Effective against various broadleaf weeds | Limited registration |
Ecotoxicological Impact | Low toxicity to mammals; irritant properties | Requires further study |
Mechanism of Action
Chlomethoxyfen acts as a protoporphyrinogen oxidase (PPO) inhibitor, which ultimately leads to the disruption of cell membranes . The inhibition of the PPO enzyme causes the accumulation of peroxidative agents that break down cell membranes, leading to cell death. This mechanism is similar to other PPO inhibitor herbicides, which are used to control broadleaf weeds .
Comparison with Similar Compounds
Structural Analogs: Diphenyl Ether Herbicides
Chlomethoxyfen belongs to the diphenyl ether herbicide class, which inhibits protoporphyrinogen oxidase (PPO), causing oxidative damage in plants. Key structural analogs include:
Table 1: Structural and Functional Comparison of Diphenyl Ether Herbicides
Key Findings:
- Antiandrogenic Activity : this compound and Chlornitrofen (CNP) exhibit stronger AR antagonism than flutamide (a pharmaceutical antiandrogen) . This activity correlates with their nitrobenzene structure, which facilitates receptor binding.
- Genotoxicity: this compound induces sister chromatid exchanges (SCEs) in V79 cells, though dose dependency is inconsistent . In contrast, Oxyfluorfen shows minimal genotoxicity, contributing to its continued use .
- Regulatory Status : this compound, CNP, and Nitrofen are banned or obsolete due to health risks, while Oxyfluorfen remains approved for specific applications .
Functional Analogs: Antiandrogenic Pesticides
This compound shares functional similarities with non-diphenyl ether compounds exhibiting endocrine-disrupting effects:
Table 2: Comparison with Antiandrogenic Pesticides
Key Findings:
- This compound’s nitrobenzene group is critical for AR antagonism, a feature shared with Fenitrothion .
- Unlike organophosphates, this compound lacks acetylcholinesterase inhibition but poses unique endocrine risks .
Environmental and Agricultural Behavior
Biological Activity
Chlomethoxyfen is a herbicide belonging to the chemical class of diphenyl ethers, primarily used for controlling broadleaf weeds in various crops. Its biological activity is characterized by its mode of action, toxicological effects, and environmental impact. This article explores these aspects in detail, supported by data tables and relevant studies.
1. Mode of Action
This compound acts primarily by inhibiting the enzyme phenylalanine ammonia-lyase (PAL) in plants. This inhibition leads to the accumulation of phenolic compounds, which are crucial for plant defense mechanisms. The increase in biphenyl-2-ol content enhances the plant's resistance to pathogens and pests.
Table 1: Mode of Action of this compound
Mechanism | Description |
---|---|
Enzyme Inhibition | Inhibits phenylalanine ammonia-lyase (PAL) |
Compound Accumulation | Increases levels of biphenyl-2-ol |
Result | Enhanced plant resistance to pathogens |
2. Toxicological Profile
This compound exhibits various toxicological effects on non-target organisms, including mammals and aquatic life. Studies have shown that it can disrupt endocrine functions and may have carcinogenic potential.
Case Study: Endocrine Disruption
A study highlighted the endocrine-disrupting effects of related herbicides like oxyfluorfen, suggesting similar risks for this compound due to structural similarities. In vitro assays indicated that exposure could lead to altered hormone levels and reproductive issues in exposed organisms .
Table 2: Toxicological Effects of this compound
Organism Type | Effect | Reference |
---|---|---|
Mammals | Hormonal imbalance | |
Aquatic Life | Behavioral changes | |
Plants | Enhanced disease resistance |
3. Environmental Impact
Table 3: Environmental Persistence
Property | Value |
---|---|
Bioaccumulation Potential | Low |
Environmental Half-life | Moderate (varies by conditions) |
4. Research Findings
Recent studies have investigated the effects of this compound on various biological systems:
- Cell Viability Studies : Research demonstrated that this compound affects cell viability in certain bacterial strains, indicating potential impacts on microbial communities in agricultural soils .
- Plant Growth Studies : Experiments showed that while this compound can enhance certain plant defenses, it may also inhibit growth under specific conditions .
5. Conclusion
This compound exhibits significant biological activity through its mode of action as a herbicide, impacting both target and non-target organisms. Its potential for endocrine disruption and environmental persistence necessitates careful consideration in agricultural practices. Future research should focus on long-term ecological impacts and the development of safer alternatives.
Q & A
Basic Research Questions
Q. What are the critical steps in synthesizing Chlomethoxyfen, and how can researchers optimize reaction yields?
this compound is synthesized via a two-step process:
- Step 1 : Reacting 2,4-dichlorophenol with 5-chloro-2-nitrophenol in the presence of NaOH and DMSO to form 2,4-dichloro-1-(3-hydroxy-4-nitrophenoxy)benzene.
- Step 2 : Methylation of the intermediate using methyl bromide . To optimize yields, researchers should monitor reaction parameters (e.g., solvent purity, temperature control) and validate intermediate purity via HPLC or NMR before proceeding to methylation.
Q. How can this compound be reliably identified and quantified in environmental samples?
Use high-performance liquid chromatography (HPLC) coupled with mass spectrometry (MS) for trace detection. The CAS RN 32861-85-1 ensures unambiguous identification in databases like SciFinder . For quantification, prepare calibration curves using this compound standard solutions (e.g., 10 mg/L in acetonitrile), validated for pesticide residue analysis .
Q. What are the primary metabolic pathways of this compound in soil, and how do they influence experimental design?
this compound degrades via microbial nitro-reduction and ether cleavage. Design experiments to measure half-life under varying soil pH and microbial activity. Include controls with sterile soil to isolate abiotic degradation pathways .
Advanced Research Questions
Q. How do contradictory findings in this compound’s genotoxicity studies (e.g., sister chromatid exchange vs. negative rec assay results) inform risk assessment?
While this compound induces sister chromatid exchanges in V79 cells, poor dose dependency and lack of rec assay positivity suggest non-DNA-reactive mechanisms (e.g., oxidative stress). Researchers should combine in vitro assays (e.g., comet assay) with in vivo models to resolve contradictions .
Q. What methodologies are recommended for analyzing this compound’s mitotic toxicity in non-target organisms?
Use MI50/SI50 indices to quantify mitotic inhibition in cell lines. For example, compare treated and solvent-control groups using fluorescence-activated cell sorting (FACS) to assess cell cycle arrest. Validate findings with dose-response curves and statistical tests for significance .
Q. How can researchers address discrepancies in regulatory limits for this compound across jurisdictions?
Conduct meta-analyses of toxicity data (e.g., NOAEL/LOAEL) and align experimental protocols with OECD or EPA guidelines. Cross-reference ZDHC MRSL limits and advocate for harmonized standards through collaborative studies .
Q. What advanced spectroscopic techniques are suitable for characterizing this compound’s degradation byproducts?
Employ tandem MS (MS/MS) for structural elucidation of nitro-reduced metabolites. Pair with Fourier-transform infrared spectroscopy (FTIR) to identify functional groups (e.g., methoxy, nitro) in degradation products .
Q. Methodological Guidance
Q. How should researchers design studies to evaluate this compound’s herbicidal efficacy without confounding environmental variables?
- Use randomized block designs with replicates to control soil heterogeneity.
- Measure soil organic matter, moisture, and microbial biomass as covariates.
- Apply ANOVA or mixed-effects models to isolate treatment effects .
Q. What statistical approaches are robust for analyzing dose-response relationships in this compound toxicity assays?
Fit data to log-logistic models (e.g., EC50 estimation) using software like R’s drc package. Report confidence intervals and apply Benjamini-Hochberg corrections for multiple comparisons .
Q. Data Reporting and Compliance
Q. What documentation is essential for publishing this compound-related research in compliance with journal guidelines?
- Experimental Section : Detail synthesis protocols, purity validation (e.g., NMR spectra), and statistical methods.
- Supporting Information : Include raw chromatograms, dose-response curves, and CAS RN cross-references.
- Ethics Statements : Disclose compliance with institutional review boards for studies involving human/animal subjects .
Properties
IUPAC Name |
4-(2,4-dichlorophenoxy)-2-methoxy-1-nitrobenzene | |
---|---|---|
Source | PubChem | |
URL | https://pubchem.ncbi.nlm.nih.gov | |
Description | Data deposited in or computed by PubChem | |
InChI |
InChI=1S/C13H9Cl2NO4/c1-19-13-7-9(3-4-11(13)16(17)18)20-12-5-2-8(14)6-10(12)15/h2-7H,1H3 | |
Source | PubChem | |
URL | https://pubchem.ncbi.nlm.nih.gov | |
Description | Data deposited in or computed by PubChem | |
InChI Key |
DXXVCXKMSWHGTF-UHFFFAOYSA-N | |
Source | PubChem | |
URL | https://pubchem.ncbi.nlm.nih.gov | |
Description | Data deposited in or computed by PubChem | |
Canonical SMILES |
COC1=C(C=CC(=C1)OC2=C(C=C(C=C2)Cl)Cl)[N+](=O)[O-] | |
Source | PubChem | |
URL | https://pubchem.ncbi.nlm.nih.gov | |
Description | Data deposited in or computed by PubChem | |
Molecular Formula |
C13H9Cl2NO4 | |
Source | PubChem | |
URL | https://pubchem.ncbi.nlm.nih.gov | |
Description | Data deposited in or computed by PubChem | |
DSSTOX Substance ID |
DTXSID6058055 | |
Record name | Chlomethoxyfen | |
Source | EPA DSSTox | |
URL | https://comptox.epa.gov/dashboard/DTXSID6058055 | |
Description | DSSTox provides a high quality public chemistry resource for supporting improved predictive toxicology. | |
Molecular Weight |
314.12 g/mol | |
Source | PubChem | |
URL | https://pubchem.ncbi.nlm.nih.gov | |
Description | Data deposited in or computed by PubChem | |
CAS No. |
32861-85-1 | |
Record name | Chlomethoxyfen | |
Source | CAS Common Chemistry | |
URL | https://commonchemistry.cas.org/detail?cas_rn=32861-85-1 | |
Description | CAS Common Chemistry is an open community resource for accessing chemical information. Nearly 500,000 chemical substances from CAS REGISTRY cover areas of community interest, including common and frequently regulated chemicals, and those relevant to high school and undergraduate chemistry classes. This chemical information, curated by our expert scientists, is provided in alignment with our mission as a division of the American Chemical Society. | |
Explanation | The data from CAS Common Chemistry is provided under a CC-BY-NC 4.0 license, unless otherwise stated. | |
Record name | Chlomethoxyfen [BSI:ISO] | |
Source | ChemIDplus | |
URL | https://pubchem.ncbi.nlm.nih.gov/substance/?source=chemidplus&sourceid=0032861851 | |
Description | ChemIDplus is a free, web search system that provides access to the structure and nomenclature authority files used for the identification of chemical substances cited in National Library of Medicine (NLM) databases, including the TOXNET system. | |
Record name | Chlomethoxyfen | |
Source | EPA DSSTox | |
URL | https://comptox.epa.gov/dashboard/DTXSID6058055 | |
Description | DSSTox provides a high quality public chemistry resource for supporting improved predictive toxicology. | |
Record name | 2,4-dichlorophenyl 3-methoxy-4-nitrophenyl ether | |
Source | European Chemicals Agency (ECHA) | |
URL | https://echa.europa.eu/substance-information/-/substanceinfo/100.046.591 | |
Description | The European Chemicals Agency (ECHA) is an agency of the European Union which is the driving force among regulatory authorities in implementing the EU's groundbreaking chemicals legislation for the benefit of human health and the environment as well as for innovation and competitiveness. | |
Explanation | Use of the information, documents and data from the ECHA website is subject to the terms and conditions of this Legal Notice, and subject to other binding limitations provided for under applicable law, the information, documents and data made available on the ECHA website may be reproduced, distributed and/or used, totally or in part, for non-commercial purposes provided that ECHA is acknowledged as the source: "Source: European Chemicals Agency, http://echa.europa.eu/". Such acknowledgement must be included in each copy of the material. ECHA permits and encourages organisations and individuals to create links to the ECHA website under the following cumulative conditions: Links can only be made to webpages that provide a link to the Legal Notice page. | |
Record name | CHLOMETHOXYFEN | |
Source | FDA Global Substance Registration System (GSRS) | |
URL | https://gsrs.ncats.nih.gov/ginas/app/beta/substances/VO5437P1A2 | |
Description | The FDA Global Substance Registration System (GSRS) enables the efficient and accurate exchange of information on what substances are in regulated products. Instead of relying on names, which vary across regulatory domains, countries, and regions, the GSRS knowledge base makes it possible for substances to be defined by standardized, scientific descriptions. | |
Explanation | Unless otherwise noted, the contents of the FDA website (www.fda.gov), both text and graphics, are not copyrighted. They are in the public domain and may be republished, reprinted and otherwise used freely by anyone without the need to obtain permission from FDA. Credit to the U.S. Food and Drug Administration as the source is appreciated but not required. | |
Retrosynthesis Analysis
AI-Powered Synthesis Planning: Our tool employs the Template_relevance Pistachio, Template_relevance Bkms_metabolic, Template_relevance Pistachio_ringbreaker, Template_relevance Reaxys, Template_relevance Reaxys_biocatalysis model, leveraging a vast database of chemical reactions to predict feasible synthetic routes.
One-Step Synthesis Focus: Specifically designed for one-step synthesis, it provides concise and direct routes for your target compounds, streamlining the synthesis process.
Accurate Predictions: Utilizing the extensive PISTACHIO, BKMS_METABOLIC, PISTACHIO_RINGBREAKER, REAXYS, REAXYS_BIOCATALYSIS database, our tool offers high-accuracy predictions, reflecting the latest in chemical research and data.
Strategy Settings
Precursor scoring | Relevance Heuristic |
---|---|
Min. plausibility | 0.01 |
Model | Template_relevance |
Template Set | Pistachio/Bkms_metabolic/Pistachio_ringbreaker/Reaxys/Reaxys_biocatalysis |
Top-N result to add to graph | 6 |
Feasible Synthetic Routes
Disclaimer and Information on In-Vitro Research Products
Please be aware that all articles and product information presented on BenchChem are intended solely for informational purposes. The products available for purchase on BenchChem are specifically designed for in-vitro studies, which are conducted outside of living organisms. In-vitro studies, derived from the Latin term "in glass," involve experiments performed in controlled laboratory settings using cells or tissues. It is important to note that these products are not categorized as medicines or drugs, and they have not received approval from the FDA for the prevention, treatment, or cure of any medical condition, ailment, or disease. We must emphasize that any form of bodily introduction of these products into humans or animals is strictly prohibited by law. It is essential to adhere to these guidelines to ensure compliance with legal and ethical standards in research and experimentation.