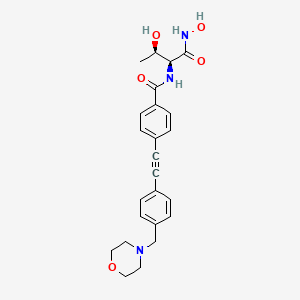
CHIR-090
Overview
Description
CHIR-090 is a potent inhibitor of the enzyme UDP-3-O-acyl-N-acetylglucosamine deacetylase (LpxC), which is crucial for the biosynthesis of lipid A, a component of the lipopolysaccharide layer in Gram-negative bacteria . This compound has shown significant antibiotic activity against various Gram-negative pathogens, including Escherichia coli and Pseudomonas aeruginosa .
Mechanism of Action
Target of Action
The primary target of CHIR-090 is the enzyme LpxC, a deacetylase involved in lipid A biosynthesis . This enzyme is essential for the survival of gram-negative bacteria, making it an attractive target for antibiotic development .
Mode of Action
This compound acts as a potent, slow, tight-binding inhibitor of the LpxC deacetylase . It binds to the enzyme with a Ki of 4.0 nM, effectively inhibiting its function . This inhibition disrupts the normal function of LpxC, which is to deacetylate UDP-3-O-[®-3-hydroxymyristoyl]-N-acetylglucosamine, a critical step in lipid A biosynthesis .
Biochemical Pathways
By inhibiting LpxC, this compound disrupts the lipid A biosynthetic pathway . Lipid A is a crucial component of the outer membrane of gram-negative bacteria, providing a protective barrier against environmental stresses . Disruption of lipid A biosynthesis can lead to bacterial cell death, making this pathway a promising target for antibiotic development .
Pharmacokinetics
It is known that this compound is a potent inhibitor of lpxc, suggesting that it has sufficient bioavailability to reach its target in bacterial cells .
Result of Action
The inhibition of LpxC by this compound leads to the disruption of lipid A biosynthesis, which can result in the death of gram-negative bacteria . This makes this compound an effective antibiotic against several gram-negative pathogens, including Pseudomonas aeruginosa and Escherichia coli .
Action Environment
It is known that this compound is effective against a range of gram-negative pathogens, suggesting that it can function in diverse environments .
Biochemical Analysis
Biochemical Properties
CHIR-090 plays a significant role in biochemical reactions, particularly in the inhibition of the LpxC deacetylase, an enzyme involved in lipid A biosynthesis . This compound binds to E. coli LpxC with a Ki of 4.0 nM . It inhibits LpxC orthologues from diverse Gram-negative pathogens, including P. aeruginosa, Neisseria meningitidis, and Helicobacter pylori .
Cellular Effects
This compound has profound effects on various types of cells and cellular processes. It influences cell function by inhibiting the LpxC deacetylase, thereby disrupting lipid A biosynthesis, an essential process in Gram-negative bacteria . This disruption can affect cell signaling pathways, gene expression, and cellular metabolism .
Molecular Mechanism
The molecular mechanism of action of this compound involves its binding to the LpxC deacetylase, inhibiting its function . This inhibition disrupts the biosynthesis of lipid A, a crucial component of the outer membrane of Gram-negative bacteria . The binding of this compound to LpxC is characterized by slow, tight-binding kinetics .
Metabolic Pathways
This compound is involved in the metabolic pathway of lipid A biosynthesis . It inhibits the LpxC deacetylase, which catalyzes the deacetylation of UDP-3-O-[®-3-hydroxymyristoyl]-N-acetylglucosamine, a key step in lipid A biosynthesis .
Preparation Methods
Synthetic Routes and Reaction Conditions
The synthesis of CHIR-090 involves the formal condensation of the carboxy group of 4-({4-[(morpholin-4-yl)methyl]phenyl}ethynyl)benzoic acid with the amino group of N-hydroxy-L-threoninamide . The reaction conditions typically include the use of coupling reagents and solvents that facilitate the formation of the amide bond.
Industrial Production Methods
While specific industrial production methods for this compound are not widely documented, the synthesis likely involves standard organic synthesis techniques, including purification steps such as crystallization or chromatography to ensure high purity of the final product .
Chemical Reactions Analysis
Types of Reactions
CHIR-090 primarily undergoes reactions typical of compounds containing hydroxamic acid and alkyne functional groups. These include:
Oxidation: The hydroxamic acid group can be oxidized under certain conditions.
Substitution: The alkyne group can participate in copper-catalyzed azide-alkyne cycloaddition (CuAAC) reactions.
Common Reagents and Conditions
Oxidation: Common oxidizing agents such as hydrogen peroxide or potassium permanganate.
Substitution: Copper(I) catalysts and azide-containing compounds for CuAAC reactions.
Major Products
The major products formed from these reactions depend on the specific reagents and conditions used. For example, CuAAC reactions yield triazole derivatives .
Scientific Research Applications
CHIR-090 has a wide range of applications in scientific research:
Comparison with Similar Compounds
Similar Compounds
L-161: Another LpxC inhibitor with different selectivity.
Ciprofloxacin: An antibiotic with a different mechanism of action but comparable antibacterial activity.
Uniqueness
CHIR-090 is unique due to its slow, tight-binding inhibition of LpxC, which results in prolonged antibacterial activity . Unlike other LpxC inhibitors, this compound has shown remarkable efficacy against a broad spectrum of Gram-negative pathogens .
Properties
IUPAC Name |
N-[(2S,3R)-3-hydroxy-1-(hydroxyamino)-1-oxobutan-2-yl]-4-[2-[4-(morpholin-4-ylmethyl)phenyl]ethynyl]benzamide | |
---|---|---|
Source | PubChem | |
URL | https://pubchem.ncbi.nlm.nih.gov | |
Description | Data deposited in or computed by PubChem | |
InChI |
InChI=1S/C24H27N3O5/c1-17(28)22(24(30)26-31)25-23(29)21-10-8-19(9-11-21)3-2-18-4-6-20(7-5-18)16-27-12-14-32-15-13-27/h4-11,17,22,28,31H,12-16H2,1H3,(H,25,29)(H,26,30)/t17-,22+/m1/s1 | |
Source | PubChem | |
URL | https://pubchem.ncbi.nlm.nih.gov | |
Description | Data deposited in or computed by PubChem | |
InChI Key |
FQYBTYFKOHPWQT-VGSWGCGISA-N | |
Source | PubChem | |
URL | https://pubchem.ncbi.nlm.nih.gov | |
Description | Data deposited in or computed by PubChem | |
Canonical SMILES |
CC(C(C(=O)NO)NC(=O)C1=CC=C(C=C1)C#CC2=CC=C(C=C2)CN3CCOCC3)O | |
Source | PubChem | |
URL | https://pubchem.ncbi.nlm.nih.gov | |
Description | Data deposited in or computed by PubChem | |
Isomeric SMILES |
C[C@H]([C@@H](C(=O)NO)NC(=O)C1=CC=C(C=C1)C#CC2=CC=C(C=C2)CN3CCOCC3)O | |
Source | PubChem | |
URL | https://pubchem.ncbi.nlm.nih.gov | |
Description | Data deposited in or computed by PubChem | |
Molecular Formula |
C24H27N3O5 | |
Source | PubChem | |
URL | https://pubchem.ncbi.nlm.nih.gov | |
Description | Data deposited in or computed by PubChem | |
DSSTOX Substance ID |
DTXSID80468361 | |
Record name | CHIR-090 | |
Source | EPA DSSTox | |
URL | https://comptox.epa.gov/dashboard/DTXSID80468361 | |
Description | DSSTox provides a high quality public chemistry resource for supporting improved predictive toxicology. | |
Molecular Weight |
437.5 g/mol | |
Source | PubChem | |
URL | https://pubchem.ncbi.nlm.nih.gov | |
Description | Data deposited in or computed by PubChem | |
CAS No. |
728865-23-4 | |
Record name | CHIR-090 | |
Source | EPA DSSTox | |
URL | https://comptox.epa.gov/dashboard/DTXSID80468361 | |
Description | DSSTox provides a high quality public chemistry resource for supporting improved predictive toxicology. | |
Retrosynthesis Analysis
AI-Powered Synthesis Planning: Our tool employs the Template_relevance Pistachio, Template_relevance Bkms_metabolic, Template_relevance Pistachio_ringbreaker, Template_relevance Reaxys, Template_relevance Reaxys_biocatalysis model, leveraging a vast database of chemical reactions to predict feasible synthetic routes.
One-Step Synthesis Focus: Specifically designed for one-step synthesis, it provides concise and direct routes for your target compounds, streamlining the synthesis process.
Accurate Predictions: Utilizing the extensive PISTACHIO, BKMS_METABOLIC, PISTACHIO_RINGBREAKER, REAXYS, REAXYS_BIOCATALYSIS database, our tool offers high-accuracy predictions, reflecting the latest in chemical research and data.
Strategy Settings
Precursor scoring | Relevance Heuristic |
---|---|
Min. plausibility | 0.01 |
Model | Template_relevance |
Template Set | Pistachio/Bkms_metabolic/Pistachio_ringbreaker/Reaxys/Reaxys_biocatalysis |
Top-N result to add to graph | 6 |
Feasible Synthetic Routes
Disclaimer and Information on In-Vitro Research Products
Please be aware that all articles and product information presented on BenchChem are intended solely for informational purposes. The products available for purchase on BenchChem are specifically designed for in-vitro studies, which are conducted outside of living organisms. In-vitro studies, derived from the Latin term "in glass," involve experiments performed in controlled laboratory settings using cells or tissues. It is important to note that these products are not categorized as medicines or drugs, and they have not received approval from the FDA for the prevention, treatment, or cure of any medical condition, ailment, or disease. We must emphasize that any form of bodily introduction of these products into humans or animals is strictly prohibited by law. It is essential to adhere to these guidelines to ensure compliance with legal and ethical standards in research and experimentation.