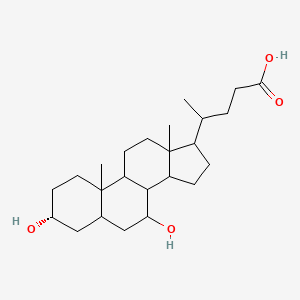
Chenodeoxycholic acid
Overview
Description
Chenodeoxycholic acid (CDCA) is a primary bile acid synthesized in the liver from cholesterol via the classic (neutral) pathway, involving 7α-hydroxylation of cholesterol by CYP7A1 . It constitutes ~30% of human bile acids and plays critical roles in lipid digestion, cholesterol homeostasis, and activation of nuclear receptors such as the farnesoid X receptor (FXR), which regulates bile acid synthesis and enterohepatic circulation . CDCA is also metabolized by gut microbiota into secondary bile acids, including lithocholic acid (LCA) and ursodeoxycholic acid (UDCA) via 7α-dehydroxylation and epimerization, respectively .
Preparation Methods
Synthetic Routes and Reaction Conditions: Chenodiol can be synthesized through various methods. One common method involves the extraction and purification of chenodeoxycholic acid from animal bile, particularly from pigs. The process includes steps such as saponification, pH regulation, decolorization, defatting, and purification using silicon gel columns . Another method involves the chemical synthesis of this compound, which includes several steps of oxidation, reduction, and crystallization .
Industrial Production Methods: Industrial production of chenodiol typically involves the extraction of this compound from animal bile, followed by purification processes to obtain a high-purity product. The production process is designed to be environmentally friendly, safe, and cost-effective, making it suitable for large-scale production .
Chemical Reactions Analysis
Types of Reactions: Chenodiol undergoes various chemical reactions, including oxidation, reduction, and substitution reactions. These reactions are essential for its synthesis and modification for therapeutic applications.
Common Reagents and Conditions: Common reagents used in the reactions involving chenodiol include oxidizing agents, reducing agents, and catalysts. The reaction conditions often involve controlled temperatures, pH levels, and specific solvents to ensure the desired chemical transformations.
Major Products Formed: These conjugates are crucial for its biological activity and therapeutic applications .
Scientific Research Applications
Treatment of Cerebrotendinous Xanthomatosis
Overview
Cerebrotendinous xanthomatosis is a rare genetic disorder caused by a deficiency in the enzyme sterol 27-hydroxylase, leading to the accumulation of cholesterol and cholestanol in various tissues. CDCA serves as a replacement therapy to facilitate the breakdown of these substances.
Clinical Evidence
A clinical evidence review indicated that treatment with CDCA significantly improved serum cholestanol levels and urinary bile alcohol levels in patients with cerebrotendinous xanthomatosis. In a retrospective cohort study involving 35 patients over an average treatment duration of 10.74 years, 100% experienced biochemical improvement, while over 70% showed stabilization or improvement in clinical symptoms .
Data Summary
Study | Patient Count | Follow-Up Duration | Key Findings |
---|---|---|---|
CDCA-STUK-15-001 | 35 | 10.74 years | Significant reduction in cholestanol levels; symptom resolution in diarrhea and cognitive impairment |
CDCA-STRCH-CR-14-001 | 28 | 5.75 years | Stabilization of disease symptoms; fewer positive outcomes compared to main study |
Del Mar Amador et al. (2018) | 14 | 5 years | Improvement in serum cholestanol; disease progression assessed |
Gallstone Dissolution
Mechanism of Action
CDCA is effective in dissolving cholesterol gallstones through its ability to reduce cholesterol saturation in bile. It alters the composition of bile, enhancing solubilization and facilitating the dissolution of gallstones.
Clinical Application
Chenodiol (a formulation of CDCA) has been utilized at a dosage of 750 mg/day for up to two years in selected patients, demonstrating effectiveness for gallstone dissolution .
Modulation of Gut Microbiome
Research Insights
Recent studies have highlighted the role of CDCA in modulating the gut microbiome, which can lead to improved overall health outcomes. The alteration of gut microbiota composition by CDCA may contribute to enhanced metabolic functions and disease resistance .
Pharmaceutical Applications in Biotechnology
Transplantation and Tissue Delivery
CDCA has been investigated for its potential applications in bio-encapsulation and transplantation, particularly concerning pancreatic islet cells for type 1 diabetes treatment. Its properties may enhance tissue delivery systems, making it a valuable compound in regenerative medicine .
Case Studies
-
Case Study on Neonatal Cholestasis
A case study reported the successful use of CDCA in a neonate presenting with cholestasis due to cerebrotendinous xanthomatosis. The early intervention led to significant clinical improvement, underscoring the importance of timely diagnosis and treatment . -
Long-term Treatment Outcomes
A longitudinal study on patients treated with CDCA for cerebrotendinous xanthomatosis revealed sustained biochemical improvements over several years, supporting its long-term efficacy and safety profile .
Safety Profile
While CDCA is generally well-tolerated, some adverse effects have been reported, including gastrointestinal disturbances and rare serious events like toxic hepatitis. Monitoring during treatment is essential to manage any potential side effects effectively .
Mechanism of Action
Chenodiol exerts its effects by suppressing the hepatic synthesis of cholesterol and cholic acid, leading to an increase in the production of cholesterol-unsaturated bile. This process helps dissolve cholesterol gallstones. Additionally, chenodiol inhibits the absorption of cholesterol in the intestines, further reducing the formation of gallstones . The molecular targets and pathways involved include the suppression of cholesterol synthesis enzymes and the modulation of bile acid transport proteins .
Comparison with Similar Compounds
Ursodeoxycholic Acid (UDCA)
UDCA, the 7β-epimer of CDCA, is a tertiary bile acid formed via microbial epimerization of CDCA in the colon .
Key Differences :
- Mechanistic Insights :
Cholic Acid (CA)
CA, another primary bile acid, shares biosynthetic pathways with CDCA but differs in hydroxylation patterns (12α-OH group in CA vs. none in CDCA).
Key Differences :
- Functional Implications :
Lithocholic Acid (LCA)
LCA, a secondary bile acid derived from CDCA via 7α-dehydroxylation, is highly toxic and carcinogenic.
Key Differences :
Hyodeoxycholic Acid (HDCA)
HDCA, a dihydroxy bile acid found in pigs, shares structural similarities with CDCA but differs in hydroxylation (6α-OH vs. 7α-OH).
Key Differences :
Parameter | CDCA | HDCA | References |
---|---|---|---|
Biosynthesis | Synthesized from cholesterol | Derived from microbial action on hyocholic acid | |
Metabolic Role | Activates FXR | Modulates glucose/lipid metabolism via TGR5 |
Enzyme Inhibition and Molecular Interactions
- AKR1D1 Inhibition: CDCA is a non-competitive inhibitor of human steroid 5β-reductase (Ki = 3.2 µM), whereas UDCA has weaker inhibitory effects (Ki = 9.8 µM) .
- RamR Binding : CDCA binds multidrug resistance regulator RamR with KD = 65.3 µM, inducing conformational changes distinct from cholic acid .
Biological Activity
Chenodeoxycholic acid (CDCA) is a primary bile acid synthesized in the liver from cholesterol. It plays a crucial role in the digestion and absorption of dietary fats and fat-soluble vitamins. Beyond its digestive functions, CDCA has significant biological activities that have been extensively studied, particularly in relation to metabolic disorders, liver diseases, and gallstone dissolution.
CDCA functions primarily by:
- Dissolving Cholesterol Gallstones : CDCA reduces cholesterol saturation in bile, facilitating the dissolution of cholesterol gallstones. It does this by promoting biliary cholesterol desaturation and inhibiting hepatic cholesterol synthesis .
- Regulating Bile Acid Synthesis : It suppresses the synthesis of both cholesterol and cholic acid in the liver, gradually replacing them with bile acids that are less harmful to liver cells .
- Modulating Gut Microbiome : Recent studies indicate that CDCA can influence gut microbiota composition, which may enhance metabolic health and reduce inflammation .
Clinical Applications
CDCA is clinically utilized for several conditions:
- Gallstone Dissolution : CDCA is effective in treating cholesterol gallstones. A study reported that patients treated with CDCA showed significant reductions in gallstone size or complete dissolution .
- Cerebrotendinous Xanthomatosis (CTX) : In patients with CTX, CDCA therapy has led to improvements in serum cholestanol levels and stabilization of neurological symptoms .
- Metabolic Disorders : Research suggests that CDCA can activate brown adipose tissue (BAT), leading to increased energy expenditure and potential benefits for obesity management .
Table 1: Summary of Clinical Studies on CDCA
Case Studies
-
Gallstone Treatment :
- A clinical trial involving 10 patients with gallstones demonstrated that administration of 1 g of CDCA daily resulted in the disappearance or reduction of stones in several cases. Liver biopsies indicated minor changes, but no serious adverse effects were reported.
-
Cerebrotendinous Xanthomatosis :
- In a retrospective analysis of patients treated with CDCA, there was a notable decrease in cholestanol levels, indicating effective control over biochemical markers associated with CTX. Additionally, clinical assessments showed stabilization or improvement in disability scores among younger patients with lower baseline disability.
Safety and Side Effects
CDCA is generally well-tolerated; however, mild-to-moderate side effects can occur, including diarrhea and abdominal discomfort. Serious adverse events are rare but can include hepatotoxicity .
Q & A
Basic Research Questions
Q. What are the primary biochemical roles of chenodeoxycholic acid in mammalian systems, and how can these be experimentally validated?
CDCA functions as a lipid emulsifier, Farnesoid X Receptor (FXR) agonist, and regulator of cholesterol homeostasis. To validate these roles:
- Use in vitro assays (e.g., luciferase reporter systems for FXR activation) and lipid solubilization tests .
- Quantify cholesterol crystallization inhibition via bile acid supersaturation models .
- Validate metabolic effects using hepatocyte cultures or animal models with bile acid sequestration techniques .
Q. What standardized analytical methods are recommended for quantifying CDCA in biological matrices?
- High-performance liquid chromatography (HPLC) with UV or mass spectrometry detection is widely used for bile acid profiling .
- Molecularly imprinted polymer (MIP)-based sensors (e.g., QCM nanosensors) offer high specificity for distinguishing CDCA from structurally similar bile acids like cholic acid .
- Enzymatic assays (e.g., 3α-hydroxysteroid dehydrogenase) can measure total bile acids but lack CDCA specificity .
Q. How is CDCA synthesized industrially, and what are the key quality control parameters?
- CDCA is primarily derived from cholic acid via 7α-dehydroxylation or microbial biotransformation. Key steps include:
- Chemical reduction of 7-keto intermediates .
- Purity validation (>95%) via HPLC and nuclear magnetic resonance (NMR) .
- Critical quality parameters: absence of lithocholic acid (hepatotoxic metabolite) and residual solvents .
Advanced Research Questions
Q. How can researchers reconcile contradictory findings on CDCA’s dual roles in cholesterol regulation (e.g., gallstone dissolution vs. bile cholesterol saturation)?
- Context-dependent analysis : CDCA reduces cholesterol saturation in human gallstones but may increase it in canine models due to species-specific bile acid metabolism .
- Methodological adjustments : Use isotopic tracer studies (e.g., deuterated cholesterol) to track cholesterol flux in vivo .
- Dose optimization : Clinical studies suggest 750 mg/day in adults balances efficacy and hepatotoxicity .
Q. What experimental strategies mitigate CDCA’s hepatotoxicity in in vitro and in vivo models?
- Conjugation approaches : Glycine or taurine conjugation reduces membrane disruption in hepatocytes .
- Targeted delivery systems : Nanoparticle encapsulation improves bile acid specificity and minimizes off-target effects .
- Co-administration with ursodeoxycholic acid (UDCA) counteracts CDCA-induced cytotoxicity .
Q. What are the challenges in designing clinical trials for CDCA in rare diseases like cerebrotendinous xanthomatosis (CTX)?
- Small cohort sizes : Retrospective multi-center collaborations are critical (e.g., European studies with n=35–28) .
- Endpoint selection : Use surrogate biomarkers (e.g., serum cholestanol) and neuroimaging to assess efficacy .
- Long-term safety monitoring : Track liver enzymes and bile acid profiles to detect subclinical hepatotoxicity .
Q. How do structural modifications of CDCA (e.g., 6α-ethyl derivatives) enhance FXR agonism, and what are the trade-offs?
- 6α-ethyl-CDCA (obeticholic acid) : Increases FXR binding affinity 100-fold but elevates pruritus risk in patients .
- Methodological insights : Molecular docking studies and X-ray crystallography reveal steric and electronic interactions at the FXR ligand-binding domain .
- Balancing potency and safety : In silico toxicity prediction models (e.g., ProTox-II) guide derivative optimization .
Q. Data Interpretation and Contradictions
Q. How should researchers address discrepancies between in vitro and in vivo CDCA efficacy data?
- Physiological relevance : In vitro models often lack bile acid enterohepatic circulation, altering exposure kinetics .
- Microbiome considerations : Gut microbiota metabolize CDCA into lithocholic acid, requiring germ-free models for mechanistic studies .
- Multi-omics integration : Pair transcriptomics (e.g., FXR target genes) with metabolomics to resolve pathway-specific effects .
Q. Why do studies report conflicting results on CDCA’s impact on intestinal barrier function?
- Dose-dependent effects : Low doses enhance barrier integrity via FXR signaling, while high doses disrupt tight junctions .
- Model variability : Use polarized epithelial cell monolayers (e.g., Caco-2) with TEER measurements for standardized assessments .
Q. Methodological Best Practices
Q. What protocols optimize CDCA stability in experimental formulations?
- Storage conditions : Lyophilized CDCA is stable at -20°C; avoid repeated freeze-thaw cycles in solution .
- Antioxidant additives : Include 0.1% ascorbic acid to prevent oxidation in cell culture media .
Q. How can researchers validate CDCA’s role in modulating gut-liver axis signaling?
Properties
IUPAC Name |
(4R)-4-[(3R,5S,7R,8R,9S,10S,13R,14S,17R)-3,7-dihydroxy-10,13-dimethyl-2,3,4,5,6,7,8,9,11,12,14,15,16,17-tetradecahydro-1H-cyclopenta[a]phenanthren-17-yl]pentanoic acid | |
---|---|---|
Source | PubChem | |
URL | https://pubchem.ncbi.nlm.nih.gov | |
Description | Data deposited in or computed by PubChem | |
InChI |
InChI=1S/C24H40O4/c1-14(4-7-21(27)28)17-5-6-18-22-19(9-11-24(17,18)3)23(2)10-8-16(25)12-15(23)13-20(22)26/h14-20,22,25-26H,4-13H2,1-3H3,(H,27,28)/t14-,15+,16-,17-,18+,19+,20-,22+,23+,24-/m1/s1 | |
Source | PubChem | |
URL | https://pubchem.ncbi.nlm.nih.gov | |
Description | Data deposited in or computed by PubChem | |
InChI Key |
RUDATBOHQWOJDD-BSWAIDMHSA-N | |
Source | PubChem | |
URL | https://pubchem.ncbi.nlm.nih.gov | |
Description | Data deposited in or computed by PubChem | |
Canonical SMILES |
CC(CCC(=O)O)C1CCC2C1(CCC3C2C(CC4C3(CCC(C4)O)C)O)C | |
Source | PubChem | |
URL | https://pubchem.ncbi.nlm.nih.gov | |
Description | Data deposited in or computed by PubChem | |
Isomeric SMILES |
C[C@H](CCC(=O)O)[C@H]1CC[C@@H]2[C@@]1(CC[C@H]3[C@H]2[C@@H](C[C@H]4[C@@]3(CC[C@H](C4)O)C)O)C | |
Source | PubChem | |
URL | https://pubchem.ncbi.nlm.nih.gov | |
Description | Data deposited in or computed by PubChem | |
Molecular Formula |
C24H40O4 | |
Source | PubChem | |
URL | https://pubchem.ncbi.nlm.nih.gov | |
Description | Data deposited in or computed by PubChem | |
DSSTOX Substance ID |
DTXSID2020260 | |
Record name | Chenodeoxycholic acid | |
Source | EPA DSSTox | |
URL | https://comptox.epa.gov/dashboard/DTXSID2020260 | |
Description | DSSTox provides a high quality public chemistry resource for supporting improved predictive toxicology. | |
Molecular Weight |
392.6 g/mol | |
Source | PubChem | |
URL | https://pubchem.ncbi.nlm.nih.gov | |
Description | Data deposited in or computed by PubChem | |
Physical Description |
Solid | |
Record name | Chenodeoxycholic acid | |
Source | Human Metabolome Database (HMDB) | |
URL | http://www.hmdb.ca/metabolites/HMDB0000518 | |
Description | The Human Metabolome Database (HMDB) is a freely available electronic database containing detailed information about small molecule metabolites found in the human body. | |
Explanation | HMDB is offered to the public as a freely available resource. Use and re-distribution of the data, in whole or in part, for commercial purposes requires explicit permission of the authors and explicit acknowledgment of the source material (HMDB) and the original publication (see the HMDB citing page). We ask that users who download significant portions of the database cite the HMDB paper in any resulting publications. | |
Solubility |
0.0899 mg/mL | |
Record name | Chenodeoxycholic acid | |
Source | DrugBank | |
URL | https://www.drugbank.ca/drugs/DB06777 | |
Description | The DrugBank database is a unique bioinformatics and cheminformatics resource that combines detailed drug (i.e. chemical, pharmacological and pharmaceutical) data with comprehensive drug target (i.e. sequence, structure, and pathway) information. | |
Explanation | Creative Common's Attribution-NonCommercial 4.0 International License (http://creativecommons.org/licenses/by-nc/4.0/legalcode) | |
Record name | Chenodeoxycholic acid | |
Source | Human Metabolome Database (HMDB) | |
URL | http://www.hmdb.ca/metabolites/HMDB0000518 | |
Description | The Human Metabolome Database (HMDB) is a freely available electronic database containing detailed information about small molecule metabolites found in the human body. | |
Explanation | HMDB is offered to the public as a freely available resource. Use and re-distribution of the data, in whole or in part, for commercial purposes requires explicit permission of the authors and explicit acknowledgment of the source material (HMDB) and the original publication (see the HMDB citing page). We ask that users who download significant portions of the database cite the HMDB paper in any resulting publications. | |
Mechanism of Action |
Chenodiol suppresses hepatic synthesis of both cholesterol and cholic acid, gradually replacing the latter and its metabolite, deoxycholic acid in an expanded bile acid pool. These actions contribute to biliary cholesterol desaturation and gradual dissolution of radiolucent cholesterol gallstones in the presence of a gall-bladder visualized by oral cholecystography. Bile acids may also bind the the bile acid receptor (FXR) which regulates the synthesis and transport of bile acids. | |
Record name | Chenodeoxycholic acid | |
Source | DrugBank | |
URL | https://www.drugbank.ca/drugs/DB06777 | |
Description | The DrugBank database is a unique bioinformatics and cheminformatics resource that combines detailed drug (i.e. chemical, pharmacological and pharmaceutical) data with comprehensive drug target (i.e. sequence, structure, and pathway) information. | |
Explanation | Creative Common's Attribution-NonCommercial 4.0 International License (http://creativecommons.org/licenses/by-nc/4.0/legalcode) | |
CAS No. |
474-25-9 | |
Record name | Chenodeoxycholic acid | |
Source | CAS Common Chemistry | |
URL | https://commonchemistry.cas.org/detail?cas_rn=474-25-9 | |
Description | CAS Common Chemistry is an open community resource for accessing chemical information. Nearly 500,000 chemical substances from CAS REGISTRY cover areas of community interest, including common and frequently regulated chemicals, and those relevant to high school and undergraduate chemistry classes. This chemical information, curated by our expert scientists, is provided in alignment with our mission as a division of the American Chemical Society. | |
Explanation | The data from CAS Common Chemistry is provided under a CC-BY-NC 4.0 license, unless otherwise stated. | |
Record name | Chenodiol [USAN] | |
Source | ChemIDplus | |
URL | https://pubchem.ncbi.nlm.nih.gov/substance/?source=chemidplus&sourceid=0000474259 | |
Description | ChemIDplus is a free, web search system that provides access to the structure and nomenclature authority files used for the identification of chemical substances cited in National Library of Medicine (NLM) databases, including the TOXNET system. | |
Record name | Chenodeoxycholic acid | |
Source | DrugBank | |
URL | https://www.drugbank.ca/drugs/DB06777 | |
Description | The DrugBank database is a unique bioinformatics and cheminformatics resource that combines detailed drug (i.e. chemical, pharmacological and pharmaceutical) data with comprehensive drug target (i.e. sequence, structure, and pathway) information. | |
Explanation | Creative Common's Attribution-NonCommercial 4.0 International License (http://creativecommons.org/licenses/by-nc/4.0/legalcode) | |
Record name | Chenodeoxycholic acid | |
Source | EPA DSSTox | |
URL | https://comptox.epa.gov/dashboard/DTXSID2020260 | |
Description | DSSTox provides a high quality public chemistry resource for supporting improved predictive toxicology. | |
Record name | Chenodeoxycholic acid | |
Source | European Chemicals Agency (ECHA) | |
URL | https://echa.europa.eu/substance-information/-/substanceinfo/100.006.803 | |
Description | The European Chemicals Agency (ECHA) is an agency of the European Union which is the driving force among regulatory authorities in implementing the EU's groundbreaking chemicals legislation for the benefit of human health and the environment as well as for innovation and competitiveness. | |
Explanation | Use of the information, documents and data from the ECHA website is subject to the terms and conditions of this Legal Notice, and subject to other binding limitations provided for under applicable law, the information, documents and data made available on the ECHA website may be reproduced, distributed and/or used, totally or in part, for non-commercial purposes provided that ECHA is acknowledged as the source: "Source: European Chemicals Agency, http://echa.europa.eu/". Such acknowledgement must be included in each copy of the material. ECHA permits and encourages organisations and individuals to create links to the ECHA website under the following cumulative conditions: Links can only be made to webpages that provide a link to the Legal Notice page. | |
Record name | CHENODIOL | |
Source | FDA Global Substance Registration System (GSRS) | |
URL | https://gsrs.ncats.nih.gov/ginas/app/beta/substances/0GEI24LG0J | |
Description | The FDA Global Substance Registration System (GSRS) enables the efficient and accurate exchange of information on what substances are in regulated products. Instead of relying on names, which vary across regulatory domains, countries, and regions, the GSRS knowledge base makes it possible for substances to be defined by standardized, scientific descriptions. | |
Explanation | Unless otherwise noted, the contents of the FDA website (www.fda.gov), both text and graphics, are not copyrighted. They are in the public domain and may be republished, reprinted and otherwise used freely by anyone without the need to obtain permission from FDA. Credit to the U.S. Food and Drug Administration as the source is appreciated but not required. | |
Record name | Chenodeoxycholic acid | |
Source | Human Metabolome Database (HMDB) | |
URL | http://www.hmdb.ca/metabolites/HMDB0000518 | |
Description | The Human Metabolome Database (HMDB) is a freely available electronic database containing detailed information about small molecule metabolites found in the human body. | |
Explanation | HMDB is offered to the public as a freely available resource. Use and re-distribution of the data, in whole or in part, for commercial purposes requires explicit permission of the authors and explicit acknowledgment of the source material (HMDB) and the original publication (see the HMDB citing page). We ask that users who download significant portions of the database cite the HMDB paper in any resulting publications. | |
Melting Point |
168-171, 165 - 167 °C | |
Record name | Chenodeoxycholic acid | |
Source | DrugBank | |
URL | https://www.drugbank.ca/drugs/DB06777 | |
Description | The DrugBank database is a unique bioinformatics and cheminformatics resource that combines detailed drug (i.e. chemical, pharmacological and pharmaceutical) data with comprehensive drug target (i.e. sequence, structure, and pathway) information. | |
Explanation | Creative Common's Attribution-NonCommercial 4.0 International License (http://creativecommons.org/licenses/by-nc/4.0/legalcode) | |
Record name | Chenodeoxycholic acid | |
Source | Human Metabolome Database (HMDB) | |
URL | http://www.hmdb.ca/metabolites/HMDB0000518 | |
Description | The Human Metabolome Database (HMDB) is a freely available electronic database containing detailed information about small molecule metabolites found in the human body. | |
Explanation | HMDB is offered to the public as a freely available resource. Use and re-distribution of the data, in whole or in part, for commercial purposes requires explicit permission of the authors and explicit acknowledgment of the source material (HMDB) and the original publication (see the HMDB citing page). We ask that users who download significant portions of the database cite the HMDB paper in any resulting publications. | |
Retrosynthesis Analysis
AI-Powered Synthesis Planning: Our tool employs the Template_relevance Pistachio, Template_relevance Bkms_metabolic, Template_relevance Pistachio_ringbreaker, Template_relevance Reaxys, Template_relevance Reaxys_biocatalysis model, leveraging a vast database of chemical reactions to predict feasible synthetic routes.
One-Step Synthesis Focus: Specifically designed for one-step synthesis, it provides concise and direct routes for your target compounds, streamlining the synthesis process.
Accurate Predictions: Utilizing the extensive PISTACHIO, BKMS_METABOLIC, PISTACHIO_RINGBREAKER, REAXYS, REAXYS_BIOCATALYSIS database, our tool offers high-accuracy predictions, reflecting the latest in chemical research and data.
Strategy Settings
Precursor scoring | Relevance Heuristic |
---|---|
Min. plausibility | 0.01 |
Model | Template_relevance |
Template Set | Pistachio/Bkms_metabolic/Pistachio_ringbreaker/Reaxys/Reaxys_biocatalysis |
Top-N result to add to graph | 6 |
Feasible Synthetic Routes
Disclaimer and Information on In-Vitro Research Products
Please be aware that all articles and product information presented on BenchChem are intended solely for informational purposes. The products available for purchase on BenchChem are specifically designed for in-vitro studies, which are conducted outside of living organisms. In-vitro studies, derived from the Latin term "in glass," involve experiments performed in controlled laboratory settings using cells or tissues. It is important to note that these products are not categorized as medicines or drugs, and they have not received approval from the FDA for the prevention, treatment, or cure of any medical condition, ailment, or disease. We must emphasize that any form of bodily introduction of these products into humans or animals is strictly prohibited by law. It is essential to adhere to these guidelines to ensure compliance with legal and ethical standards in research and experimentation.