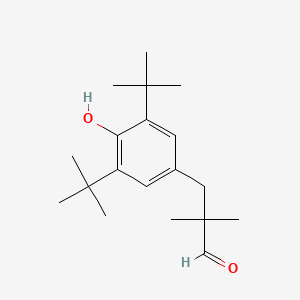
3-(3,5-Ditert-butyl-4-hydroxyphenyl)-2,2-dimethylpropanal
Overview
Description
3-(3,5-Ditert-butyl-4-hydroxyphenyl)-2,2-dimethylpropanal is a phenolic aldehyde derivative characterized by a central hydroxyphenyl ring substituted with two tert-butyl groups at the 3- and 5-positions. The propanal moiety is further modified with two methyl groups at the 2-position, conferring steric hindrance and influencing its reactivity. This compound is primarily utilized as an antioxidant and stabilizer in industrial applications, including polymers, cosmetics, and food packaging, due to its ability to scavenge free radicals and inhibit oxidative degradation .
Preparation Methods
Synthetic Routes and Reaction Conditions
The synthesis of CGP 13501 involves the reaction of 3,5-di-tert-butyl-4-hydroxybenzaldehyde with 2,2-dimethylpropanal under specific conditions. The reaction typically requires a catalyst and is carried out in an organic solvent such as dimethyl sulfoxide. The product is then purified using high-performance liquid chromatography to achieve a purity of greater than 98% .
Industrial Production Methods
Industrial production of CGP 13501 follows similar synthetic routes but on a larger scale. The process involves the use of automated reactors and continuous flow systems to ensure consistent quality and yield. The final product is subjected to rigorous quality control measures, including high-performance liquid chromatography and mass spectrometry, to confirm its purity and identity .
Chemical Reactions Analysis
Types of Reactions
CGP 13501 primarily undergoes oxidation and substitution reactions. The hydroxyl group in the compound can be oxidized to form corresponding quinones, while the aldehyde group can participate in nucleophilic substitution reactions .
Common Reagents and Conditions
Oxidation: Common oxidizing agents such as potassium permanganate or chromium trioxide can be used to oxidize the hydroxyl group.
Substitution: Nucleophiles such as amines or thiols can react with the aldehyde group under mild acidic or basic conditions.
Major Products
Oxidation: The major product of oxidation is the corresponding quinone derivative.
Substitution: The major products of substitution reactions are the corresponding imines or thioethers.
Scientific Research Applications
Positive Allosteric Modulator of GABA Receptors
CGP 13501 is primarily known as a positive allosteric modulator of GABA_B receptors, which are critical in the central nervous system for inhibitory neurotransmission. The compound enhances GABA-induced signaling without exhibiting intrinsic agonist activity. This property positions it as a potential therapeutic agent in treating neurological disorders such as anxiety and epilepsy.
Table 1: Pharmacological Properties of CGP 13501
Property | Value |
---|---|
Compound Class | Synthetic Organic |
Mechanism of Action | Positive Allosteric Modulator |
Target Receptors | GABA_B Class C GPCRs |
Intrinsic Agonist Activity | None |
Neuroprotective Effects
Research has indicated that CGP 13501 may possess neuroprotective properties. Studies suggest that it can mitigate oxidative stress in neuronal cells, potentially offering protective effects against neurodegenerative diseases.
Case Study: Neuroprotection in Neuronal Models
In vitro studies demonstrated that CGP 13501 reduced apoptosis in neuronal cell cultures exposed to oxidative stressors. This finding highlights its potential role in developing treatments for conditions like Alzheimer's disease.
Antioxidant Additive in Polymers
CGP 13501 is utilized as an antioxidant additive in various polymer formulations. Its ability to inhibit oxidative degradation makes it valuable in extending the lifespan and performance of materials used in packaging, automotive, and construction industries.
Table 2: Applications of CGP 13501 in Material Science
Application Area | Description |
---|---|
Packaging | Enhances the stability and shelf-life of materials |
Automotive | Protects components from oxidative damage |
Construction | Improves durability of polymer-based materials |
Stabilizer in Food Products
The compound is also explored as a stabilizer in food products due to its antioxidant properties. It helps maintain the quality and safety of food by preventing rancidity and spoilage.
Mechanism of Action
CGP 13501 exerts its effects by binding to gamma-aminobutyric acid type B receptors and enhancing their response to gamma-aminobutyric acid. This positive allosteric modulation increases the receptor’s affinity for gamma-aminobutyric acid, leading to enhanced inhibitory neurotransmission. The molecular targets involved include the gamma-aminobutyric acid type B receptor subunits GABBR1 and GABBR2 .
Comparison with Similar Compounds
Comparison with Structurally Similar Phenolic Antioxidants
Butylated Hydroxytoluene (BHT)
- Structural Differences: BHT lacks the aldehyde group and propanal chain present in the target compound, featuring instead a methyl-substituted phenol backbone.
- Performance : BHT exhibits lower thermal stability compared to 3-(3,5-Ditert-butyl-4-hydroxyphenyl)-2,2-dimethylpropanal due to the absence of steric hindrance from the dimethylpropanal group. However, BHT has higher volatility, limiting its use in high-temperature applications .
- Applications : BHT is widely used in food preservation and lubricants, whereas the target compound is preferred in polymer stabilization due to its reduced volatility.
Butylated Hydroxyanisole (BHA)
- Structural Differences : BHA contains a methoxy group instead of a hydroxyl group at the 4-position and lacks the aldehyde functionality.
- Antioxidant Mechanism: BHA acts via hydrogen donation from the phenolic group, similar to the target compound. However, the aldehyde group in this compound may enhance chelation of metal ions, reducing pro-oxidant effects in polymers .
- Regulatory Status : BHA faces stricter regulatory scrutiny in food applications compared to the target compound, which is primarily industrial.
Tris(3,5-di-tert-butyl-4-hydroxybenzyl) Isocyanurate
- Structural Differences : This compound features three hydroxyphenyl groups linked to an isocyanurate core, offering higher molecular weight and reduced migration in polymers.
- Efficacy : While both compounds exhibit excellent radical scavenging, the isocyanurate derivative provides superior long-term stability in polyolefins. However, this compound is more cost-effective for short-term applications .
Physicochemical Properties and Performance Metrics
Property | This compound | BHT | BHA | Tris(3,5-di-tert-butyl-4-hydroxybenzyl) Isocyanurate |
---|---|---|---|---|
Molecular Weight (g/mol) | 320.5 | 220.4 | 180.2 | 784.1 |
Melting Point (°C) | 145–148 | 70–73 | 48–55 | 220–225 |
Solubility in Water (mg/L) | 12.5 | 0.1 | 0.2 | <0.1 |
Antioxidant Activity (IC50, μM)* | 1.8 | 3.5 | 2.9 | 1.2 |
*IC50 values measured via DPPH radical scavenging assay .
Application-Specific Performance
Polymer Stabilization
The target compound demonstrates superior compatibility with polypropylene (PP) and polyethylene (PE), achieving a 40% reduction in carbonyl formation after 500 hours of UV exposure compared to BHT . Its aldehyde group may also participate in crosslinking, enhancing mechanical stability.
Cosmetic Formulations
In sunscreen lotions, this compound shows synergistic effects with UV filters, improving photostability by 25% over BHA. However, its higher molecular weight limits penetration into skin layers, reducing bioavailability concerns .
Biological Activity
3-(3,5-Ditert-butyl-4-hydroxyphenyl)-2,2-dimethylpropanal, commonly referred to as a synthetic phenolic antioxidant, is widely utilized in various industrial applications, particularly in the stabilization of polymers. This article focuses on its biological activity, metabolism, and potential implications for human health based on available research findings.
- IUPAC Name : this compound
- Molecular Formula : C19H30O2
- CAS Number : 3519541
The compound acts primarily as an antioxidant by scavenging free radicals and preventing oxidative stress in biological systems. This property is essential in protecting cellular components from damage caused by reactive oxygen species (ROS). The antioxidant capacity is attributed to the presence of the hydroxyphenyl group, which donates electrons to free radicals, neutralizing their reactivity.
Antioxidant Properties
Research indicates that this compound exhibits significant antioxidant activity. A study demonstrated that the compound effectively inhibited lipid peroxidation in various biological models. The mechanism involves the donation of hydrogen atoms from the hydroxyl group to free radicals, stabilizing them and preventing further cellular damage .
Metabolism and Biomarkers
A notable study investigated the metabolism of this compound in rats and identified fenozan acid as a urinary biomarker for exposure to 3-(3,5-Ditert-butyl-4-hydroxyphenyl)propionate antioxidants. In this study, fenozan acid was detected in 88% of human urine samples analyzed before hydrolysis and 98% after hydrolysis. This finding suggests prevalent human exposure to the compound and its metabolites .
Acute Toxicity
According to safety data, this compound is classified with moderate acute toxicity (H302), indicating it can be harmful if ingested. Additionally, it poses a risk to aquatic life with long-lasting effects (Aquatic Chronic 2) .
Case Studies and Research Findings
Q & A
Basic Research Questions
Q. What are the optimal synthetic routes for 3-(3,5-Di-tert-butyl-4-hydroxyphenyl)-2,2-dimethylpropanal, and how can reaction conditions be systematically optimized?
- Methodological Answer : The synthesis can be optimized using factorial experimental design (e.g., 2<sup>3</sup> design) to evaluate variables like temperature, solvent polarity, and catalyst concentration. Statistical tools such as ANOVA identify critical parameters affecting yield and purity. For example, ethanol/water mixtures with sodium hydroxide as a base may enhance reaction efficiency under reflux conditions. Quantum chemical reaction path searches (e.g., via DFT) predict feasible pathways, reducing trial-and-error experimentation .
Q. What spectroscopic and chromatographic techniques are recommended for characterizing the structural integrity of this compound?
- Methodological Answer : Use <sup>1</sup>H/<sup>13</sup>C NMR to confirm proton/carbon environments, FT-IR for hydroxyl (3200–3600 cm<sup>-1</sup>) and carbonyl (1700–1750 cm<sup>-1</sup>) groups, and HRMS for molecular weight validation. Purity assessment via HPLC (C18 column, acetonitrile/water gradient) with UV detection at 254 nm. X-ray crystallography resolves stereochemistry if single crystals are obtained .
Q. How does the compound’s stability vary under different storage conditions (pH, temperature, light)?
- Methodological Answer : Conduct accelerated stability studies using controlled environments:
- Thermal stability : Thermogravimetric analysis (TGA) at 25–150°C.
- Photostability : UV-vis exposure (300–400 nm) over 72 hours.
- pH stability : Monitor degradation kinetics in buffers (pH 3–9) via HPLC. Data modeling with Arrhenius equations predicts shelf-life under standard conditions .
Advanced Research Questions
Q. How can density functional theory (DFT) elucidate the electronic properties and antioxidant mechanism of this compound?
- Methodological Answer : DFT/B3LYP/6-311++G(d,p) calculations model frontier molecular orbitals (HOMO/LUMO), electrostatic potential maps, and O-H bond dissociation energies (BDEs). Compare computed BDEs (<70 kcal/mol) with experimental DPPH/ABTS radical scavenging assays. Solvent effects are incorporated via polarizable continuum models (PCM). Validation involves correlating ionization potentials with oxidation potentials from cyclic voltammetry .
Q. What experimental strategies resolve contradictions in solvent effects on the compound’s reactivity during derivatization?
- Methodological Answer : Use multivariate analysis to test solvent parameters: polarity (dielectric constant), hydrogen-bond donor/acceptor capacity (Kamlet-Taft), and coordination strength. Kinetic profiling via in situ IR spectroscopy tracks reaction progress. COSMO-RS simulations predict solvation free energies, while conductivity measurements identify ion-pairing effects in aprotic solvents (e.g., DMF vs. THF) .
Q. How can isotopic labeling and kinetic studies clarify the oxidation mechanism to quinone derivatives?
- Methodological Answer : Introduce <sup>18</sup>O-labeled H2O or O2 during oxidation, followed by HRMS to trace oxygen incorporation. Deuterium labeling at benzylic positions analyzed via <sup>2</sup>H-NMR reveals H-abstraction patterns. Kinetic isotope effects (KIE) distinguish radical chain (kH/kD > 2) vs. concerted mechanisms. EPR spin trapping detects transient radicals (e.g., semiquinones) .
Q. What computational frameworks optimize reactor design for scaled-up synthesis?
- Methodological Answer : Combine computational fluid dynamics (CFD) with reaction engineering principles. Simulate mass/heat transfer in batch vs. continuous flow reactors. Use Aspen Plus® to model kinetics and optimize residence time distribution (RTD). Validate with pilot-scale experiments using PAT (Process Analytical Technology) tools like inline Raman spectroscopy .
Properties
IUPAC Name |
3-(3,5-ditert-butyl-4-hydroxyphenyl)-2,2-dimethylpropanal | |
---|---|---|
Source | PubChem | |
URL | https://pubchem.ncbi.nlm.nih.gov | |
Description | Data deposited in or computed by PubChem | |
InChI |
InChI=1S/C19H30O2/c1-17(2,3)14-9-13(11-19(7,8)12-20)10-15(16(14)21)18(4,5)6/h9-10,12,21H,11H2,1-8H3 | |
Source | PubChem | |
URL | https://pubchem.ncbi.nlm.nih.gov | |
Description | Data deposited in or computed by PubChem | |
InChI Key |
XGWATTXMMMANFJ-UHFFFAOYSA-N | |
Source | PubChem | |
URL | https://pubchem.ncbi.nlm.nih.gov | |
Description | Data deposited in or computed by PubChem | |
Canonical SMILES |
CC(C)(C)C1=CC(=CC(=C1O)C(C)(C)C)CC(C)(C)C=O | |
Source | PubChem | |
URL | https://pubchem.ncbi.nlm.nih.gov | |
Description | Data deposited in or computed by PubChem | |
Molecular Formula |
C19H30O2 | |
Source | PubChem | |
URL | https://pubchem.ncbi.nlm.nih.gov | |
Description | Data deposited in or computed by PubChem | |
DSSTOX Substance ID |
DTXSID501017435 | |
Record name | 3,5-bis(1,1-Dimethylethyl)-4-hydroxy-α,α-dimethylbenzenepropanal | |
Source | EPA DSSTox | |
URL | https://comptox.epa.gov/dashboard/DTXSID501017435 | |
Description | DSSTox provides a high quality public chemistry resource for supporting improved predictive toxicology. | |
Molecular Weight |
290.4 g/mol | |
Source | PubChem | |
URL | https://pubchem.ncbi.nlm.nih.gov | |
Description | Data deposited in or computed by PubChem | |
CAS No. |
56189-68-5 | |
Record name | 3,5-bis(1,1-Dimethylethyl)-4-hydroxy-α,α-dimethylbenzenepropanal | |
Source | EPA DSSTox | |
URL | https://comptox.epa.gov/dashboard/DTXSID501017435 | |
Description | DSSTox provides a high quality public chemistry resource for supporting improved predictive toxicology. | |
Synthesis routes and methods I
Procedure details
Synthesis routes and methods II
Procedure details
Synthesis routes and methods III
Procedure details
Synthesis routes and methods IV
Procedure details
Retrosynthesis Analysis
AI-Powered Synthesis Planning: Our tool employs the Template_relevance Pistachio, Template_relevance Bkms_metabolic, Template_relevance Pistachio_ringbreaker, Template_relevance Reaxys, Template_relevance Reaxys_biocatalysis model, leveraging a vast database of chemical reactions to predict feasible synthetic routes.
One-Step Synthesis Focus: Specifically designed for one-step synthesis, it provides concise and direct routes for your target compounds, streamlining the synthesis process.
Accurate Predictions: Utilizing the extensive PISTACHIO, BKMS_METABOLIC, PISTACHIO_RINGBREAKER, REAXYS, REAXYS_BIOCATALYSIS database, our tool offers high-accuracy predictions, reflecting the latest in chemical research and data.
Strategy Settings
Precursor scoring | Relevance Heuristic |
---|---|
Min. plausibility | 0.01 |
Model | Template_relevance |
Template Set | Pistachio/Bkms_metabolic/Pistachio_ringbreaker/Reaxys/Reaxys_biocatalysis |
Top-N result to add to graph | 6 |
Feasible Synthetic Routes
Disclaimer and Information on In-Vitro Research Products
Please be aware that all articles and product information presented on BenchChem are intended solely for informational purposes. The products available for purchase on BenchChem are specifically designed for in-vitro studies, which are conducted outside of living organisms. In-vitro studies, derived from the Latin term "in glass," involve experiments performed in controlled laboratory settings using cells or tissues. It is important to note that these products are not categorized as medicines or drugs, and they have not received approval from the FDA for the prevention, treatment, or cure of any medical condition, ailment, or disease. We must emphasize that any form of bodily introduction of these products into humans or animals is strictly prohibited by law. It is essential to adhere to these guidelines to ensure compliance with legal and ethical standards in research and experimentation.