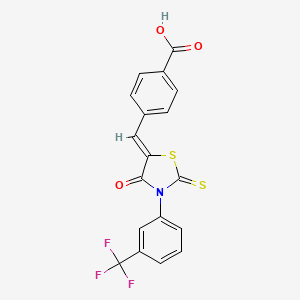
CFTR(inh)-172
Overview
Description
CFTRinh-172 is a small molecule inhibitor of the cystic fibrosis transmembrane conductance regulator (CFTR) chloride channel. This compound is widely used in scientific research to study the function and regulation of CFTR, which plays a crucial role in maintaining electrolyte and fluid balance in epithelial tissues. CFTRinh-172 has been developed as a potential therapeutic agent for conditions such as secretory diarrhea and autosomal dominant polycystic kidney disease.
Mechanism of Action
Target of Action
CFTR(inh)-172, also known as CFTRinh-172, primarily targets the Cystic Fibrosis Transmembrane Conductance Regulator (CFTR) . CFTR is a chloride channel that regulates ion and fluid transport across various epithelia . It plays a critical role in maintaining the balance of salt and water on many surfaces in the body, such as the surface of the lung .
Mode of Action
This compound acts as an inhibitor of the CFTR protein It is known that normal cftr facilitates the transport of adenosine triphosphate (atp), an energy-carrying molecule, to the outside of the cell . It is likely that this compound interferes with this process, thereby inhibiting the function of the CFTR protein.
Biochemical Pathways
The CFTR protein is involved in several biochemical pathways. It plays a crucial role in regulating transepithelial movement of water and electrolyte in exocrine tissues . Dysfunction of the CFTR protein due to mutations results in diseases like Cystic Fibrosis (CF) . This compound, by inhibiting the CFTR protein, can affect these pathways and their downstream effects.
Pharmacokinetics
It is known that the pharmacokinetics of other cftr modulators can be suboptimal . For example, ivacaftor, a CFTR potentiator, exhibits suboptimal physical properties and pharmacokinetics and is associated with side effects
Result of Action
The molecular and cellular effects of this compound’s action are primarily related to its inhibitory effect on the CFTR protein. By inhibiting the CFTR protein, this compound can disrupt the balance of salt and water on various surfaces in the body, leading to the trapping of chloride in cells . This can result in a range of effects, depending on the specific tissues affected.
Action Environment
The action, efficacy, and stability of this compound can be influenced by various environmental factors. For example, the presence of other drugs, the patient’s overall health status, and even the patient’s diet can potentially affect the action of this compound . Additionally, genetic factors, such as the presence of certain mutations in the CFTR gene, can also influence the efficacy of this compound .
Biochemical Analysis
Biochemical Properties
CFTR(inh)-172 interacts with the CFTR protein, a low-abundance membrane protein . The CFTR protein is a channel that sits on the surface of cells and transports chloride and other molecules, such as bicarbonate . The dysfunction of CFTR protein is the fundamental cause of cystic fibrosis (CF), a fatal genetic disease .
Cellular Effects
This compound influences cell function by interacting with the CFTR protein. The CFTR protein is expressed in epithelial cells throughout the body, including the lung, pancreas, and gastrointestinal tract, where it is localized in the apical membrane . There, CFTR acts as an anion channel, controlling primarily the flux of chloride and bicarbonate ions .
Molecular Mechanism
The molecular mechanism of this compound involves its interaction with the CFTR protein. The CFTR protein is a chloride and bicarbonate channel responsible for regulation of ion transport across the apical membrane at the surface of certain epithelia . The most common disease-causing mutation is F508del – the deletion of three nucleotides that leads to the deletion of the phenylalanine residue at position 508 in the polypeptidic chain .
Temporal Effects in Laboratory Settings
In laboratory settings, the effects of this compound on cellular function can be observed over time. For instance, the novel messenger RNA (mRNA)-based therapy shows high potential to treat CF disease, by delivering CFTR mRNA into lung epithelial cells to generate fully functional CFTR replacement protein .
Subcellular Localization
The subcellular localization of this compound is associated with the CFTR protein. The CFTR protein is localized in the apical membrane of epithelial cells
For a more comprehensive understanding, please refer to the original research articles and reviews .
Preparation Methods
Synthetic Routes and Reaction Conditions
The synthesis of CFTRinh-172 involves multiple steps, starting from commercially available starting materials. The key steps include the formation of a thiazolidinone ring and the introduction of a trifluoromethyl group. The reaction conditions typically involve the use of organic solvents, such as dimethyl sulfoxide (DMSO), and catalysts to facilitate the formation of the desired product.
Industrial Production Methods
Industrial production of CFTRinh-172 follows similar synthetic routes but on a larger scale. The process involves optimizing reaction conditions to maximize yield and purity. The compound is typically purified using techniques such as recrystallization or chromatography to ensure high-quality product suitable for research and potential therapeutic use.
Chemical Reactions Analysis
Types of Reactions
CFTRinh-172 undergoes various chemical reactions, including:
Oxidation: The compound can be oxidized under specific conditions to form oxidized derivatives.
Reduction: Reduction reactions can be used to modify the functional groups present in CFTRinh-172.
Substitution: The compound can undergo substitution reactions where specific functional groups are replaced with others.
Common Reagents and Conditions
Oxidation: Common oxidizing agents include hydrogen peroxide and potassium permanganate.
Reduction: Reducing agents such as sodium borohydride and lithium aluminum hydride are used.
Substitution: Reagents like halogens and nucleophiles are employed in substitution reactions.
Major Products Formed
The major products formed from these reactions depend on the specific conditions and reagents used. For example, oxidation may yield oxidized thiazolidinone derivatives, while reduction can produce reduced forms of the compound with modified functional groups.
Scientific Research Applications
CFTRinh-172 has a wide range of scientific research applications, including:
Chemistry: Used to study the chemical properties and reactivity of CFTR inhibitors.
Biology: Employed in research to understand the role of CFTR in cellular processes and its regulation.
Medicine: Investigated as a potential therapeutic agent for treating conditions like secretory diarrhea and autosomal dominant polycystic kidney disease.
Industry: Utilized in the development of new CFTR inhibitors and related compounds for therapeutic use.
Comparison with Similar Compounds
CFTRinh-172 is compared with other CFTR inhibitors, such as GlyH-101 and thiazolidinone derivatives. While all these compounds inhibit CFTR function, CFTRinh-172 is unique in its dual role as both a pore blocker and gating modulator . This distinct mechanism of action provides a molecular basis for its specificity and efficacy in inhibiting CFTR.
List of Similar Compounds
- GlyH-101
- Thiazolidinone derivatives
CFTRinh-172’s unique properties and wide range of applications make it a valuable tool in scientific research and potential therapeutic development.
Properties
IUPAC Name |
4-[[4-oxo-2-sulfanylidene-3-[3-(trifluoromethyl)phenyl]-1,3-thiazolidin-5-ylidene]methyl]benzoic acid | |
---|---|---|
Details | Computed by Lexichem TK 2.7.0 (PubChem release 2021.05.07) | |
Source | PubChem | |
URL | https://pubchem.ncbi.nlm.nih.gov | |
Description | Data deposited in or computed by PubChem | |
InChI |
InChI=1S/C18H10F3NO3S2/c19-18(20,21)12-2-1-3-13(9-12)22-15(23)14(27-17(22)26)8-10-4-6-11(7-5-10)16(24)25/h1-9H,(H,24,25) | |
Details | Computed by InChI 1.0.6 (PubChem release 2021.05.07) | |
Source | PubChem | |
URL | https://pubchem.ncbi.nlm.nih.gov | |
Description | Data deposited in or computed by PubChem | |
InChI Key |
JIMHYXZZCWVCMI-UHFFFAOYSA-N | |
Details | Computed by InChI 1.0.6 (PubChem release 2021.05.07) | |
Source | PubChem | |
URL | https://pubchem.ncbi.nlm.nih.gov | |
Description | Data deposited in or computed by PubChem | |
Canonical SMILES |
C1=CC(=CC(=C1)N2C(=O)C(=CC3=CC=C(C=C3)C(=O)O)SC2=S)C(F)(F)F | |
Details | Computed by OEChem 2.3.0 (PubChem release 2021.05.07) | |
Source | PubChem | |
URL | https://pubchem.ncbi.nlm.nih.gov | |
Description | Data deposited in or computed by PubChem | |
Molecular Formula |
C18H10F3NO3S2 | |
Details | Computed by PubChem 2.1 (PubChem release 2021.05.07) | |
Source | PubChem | |
URL | https://pubchem.ncbi.nlm.nih.gov | |
Description | Data deposited in or computed by PubChem | |
DSSTOX Substance ID |
DTXSID501317499 | |
Record name | CFTR(inh)-172 | |
Source | EPA DSSTox | |
URL | https://comptox.epa.gov/dashboard/DTXSID501317499 | |
Description | DSSTox provides a high quality public chemistry resource for supporting improved predictive toxicology. | |
Molecular Weight |
409.4 g/mol | |
Details | Computed by PubChem 2.1 (PubChem release 2021.05.07) | |
Source | PubChem | |
URL | https://pubchem.ncbi.nlm.nih.gov | |
Description | Data deposited in or computed by PubChem | |
CAS No. |
307510-92-5 | |
Record name | CFTR(inh)-172 | |
Source | CAS Common Chemistry | |
URL | https://commonchemistry.cas.org/detail?cas_rn=307510-92-5 | |
Description | CAS Common Chemistry is an open community resource for accessing chemical information. Nearly 500,000 chemical substances from CAS REGISTRY cover areas of community interest, including common and frequently regulated chemicals, and those relevant to high school and undergraduate chemistry classes. This chemical information, curated by our expert scientists, is provided in alignment with our mission as a division of the American Chemical Society. | |
Explanation | The data from CAS Common Chemistry is provided under a CC-BY-NC 4.0 license, unless otherwise stated. | |
Record name | CFTR(inh)-172 | |
Source | EPA DSSTox | |
URL | https://comptox.epa.gov/dashboard/DTXSID501317499 | |
Description | DSSTox provides a high quality public chemistry resource for supporting improved predictive toxicology. | |
Record name | 5-[(4-Carboxyphenyl)methylene]-2-thioxo-3-[(3-trifluoromethyl)phenyl-4-thiazolidinone | |
Source | European Chemicals Agency (ECHA) | |
URL | https://echa.europa.eu/information-on-chemicals | |
Description | The European Chemicals Agency (ECHA) is an agency of the European Union which is the driving force among regulatory authorities in implementing the EU's groundbreaking chemicals legislation for the benefit of human health and the environment as well as for innovation and competitiveness. | |
Explanation | Use of the information, documents and data from the ECHA website is subject to the terms and conditions of this Legal Notice, and subject to other binding limitations provided for under applicable law, the information, documents and data made available on the ECHA website may be reproduced, distributed and/or used, totally or in part, for non-commercial purposes provided that ECHA is acknowledged as the source: "Source: European Chemicals Agency, http://echa.europa.eu/". Such acknowledgement must be included in each copy of the material. ECHA permits and encourages organisations and individuals to create links to the ECHA website under the following cumulative conditions: Links can only be made to webpages that provide a link to the Legal Notice page. | |
Retrosynthesis Analysis
AI-Powered Synthesis Planning: Our tool employs the Template_relevance Pistachio, Template_relevance Bkms_metabolic, Template_relevance Pistachio_ringbreaker, Template_relevance Reaxys, Template_relevance Reaxys_biocatalysis model, leveraging a vast database of chemical reactions to predict feasible synthetic routes.
One-Step Synthesis Focus: Specifically designed for one-step synthesis, it provides concise and direct routes for your target compounds, streamlining the synthesis process.
Accurate Predictions: Utilizing the extensive PISTACHIO, BKMS_METABOLIC, PISTACHIO_RINGBREAKER, REAXYS, REAXYS_BIOCATALYSIS database, our tool offers high-accuracy predictions, reflecting the latest in chemical research and data.
Strategy Settings
Precursor scoring | Relevance Heuristic |
---|---|
Min. plausibility | 0.01 |
Model | Template_relevance |
Template Set | Pistachio/Bkms_metabolic/Pistachio_ringbreaker/Reaxys/Reaxys_biocatalysis |
Top-N result to add to graph | 6 |
Feasible Synthetic Routes
Disclaimer and Information on In-Vitro Research Products
Please be aware that all articles and product information presented on BenchChem are intended solely for informational purposes. The products available for purchase on BenchChem are specifically designed for in-vitro studies, which are conducted outside of living organisms. In-vitro studies, derived from the Latin term "in glass," involve experiments performed in controlled laboratory settings using cells or tissues. It is important to note that these products are not categorized as medicines or drugs, and they have not received approval from the FDA for the prevention, treatment, or cure of any medical condition, ailment, or disease. We must emphasize that any form of bodily introduction of these products into humans or animals is strictly prohibited by law. It is essential to adhere to these guidelines to ensure compliance with legal and ethical standards in research and experimentation.