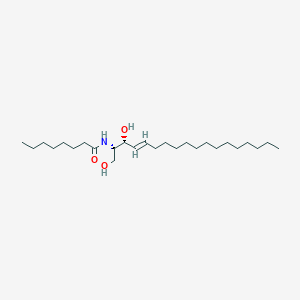
C8 ceramide
Overview
Description
C8-Ceramide, also known as N-octanoyl-D-erythro-sphingosine, is a synthetic analog of naturally occurring ceramides. Ceramides are a family of lipid molecules composed of sphingosine and a fatty acid. They play a crucial role in cellular signaling, particularly in the regulation of cell growth, differentiation, and apoptosis. C8-Ceramide has gained significant attention in scientific research due to its potent biological activities and potential therapeutic applications.
Scientific Research Applications
C8-Ceramide has a wide range of scientific research applications, including:
Chemistry: Used as a model compound to study the behavior of ceramides in various chemical reactions.
Biology: Investigated for its role in cellular signaling pathways, particularly in apoptosis and cell differentiation.
Medicine: Explored as a potential therapeutic agent for cancer treatment due to its ability to induce apoptosis in cancer cells
Industry: Utilized in the development of drug delivery systems and as a component in cosmetic formulations.
Mechanism of Action
Target of Action
C8 Ceramide, also known as N-octanoylsphingosine, C8-ceramide, or C-8 Ceramide, is a biologically active ceramide analog . It has been shown to have potent anti-proliferative effects against cancer cells . The primary targets of this compound are hepatocellular carcinoma cells and non-small-cell lung cancer cells . It also targets microglia, the resident immune cells in the central nervous system .
Mode of Action
This compound interacts with its targets by inducing apoptosis and inhibiting cell proliferation . It activates caspase-dependent apoptosis in hepatocellular carcinoma cells . The cytotoxicity of this compound is significantly attenuated with co-treatment of caspase inhibitors . It also increases the level of endogenous reactive oxygen species (ROS) in non-small-cell lung cancer cells .
Biochemical Pathways
This compound affects several biochemical pathways. It activates ASK1 (apoptosis signal-regulating kinase 1)-JNK (Jun N-terminal protein kinase) signaling in hepatocellular carcinoma cells . It also inhibits AKT-mTOR (mammalian target of rapamycin) activation in these cells . In microglia, this compound up-regulates the expression of BDNF mRNA after being treated with lipopolysaccharide (LPS) in vitro . This effect is mediated via the PKCδ/NF-κB signaling pathway .
Pharmacokinetics
This compound plays a structural role in liposome formulations and enhances the cellular uptake of amphiphilic drugs, such as chemotherapies . It appears to enhance the bioavailability of drugs by acting as a lipid delivery system .
Result of Action
The result of this compound’s action is the inhibition of cell survival and proliferation, and the induction of apoptosis . In hepatocellular carcinoma cells, it leads to caspase-dependent apoptosis . In non-small-cell lung cancer cells, it increases the level of endogenous ROS . In microglia, it up-regulates the expression of BDNF mRNA .
Action Environment
The action of this compound can be influenced by environmental factors. For instance, lipopolysaccharide (LPS) treatment in vitro stimulates primary microglia to up-regulate the expression of BDNF mRNA . Furthermore, the efficacy of this compound in improving postoperative cognitive dysfunction in mice was observed after carotid artery exposure .
Safety and Hazards
Biochemical Analysis
Biochemical Properties
C8 Ceramide interacts with several enzymes and proteins, playing a significant role in biochemical reactions . It is metabolized within cells to generate natural ceramides, often causing a dramatic increase in cellular ceramide levels . This process involves the acylation of sphinganine to dihydroceramide, which is subsequently reduced to ceramide .
Cellular Effects
This compound has broad effects on different types of cells, including endothelial cells, microglia, and neurons . It has been shown to increase the level of endogenous reactive oxygen species (ROS) in human non-small-cell lung cancer H1299 cells . Furthermore, it has been demonstrated to induce apoptosis and have anti-proliferative properties .
Molecular Mechanism
This compound exerts its effects at the molecular level through various mechanisms. It has been shown to enhance the cognitive function of mice via the PKCδ/NF-κB signaling pathway . Furthermore, it stimulates primary microglia to up-regulate the expression of BDNF mRNA after being treated with lipopolysaccharide (LPS) in vitro .
Temporal Effects in Laboratory Settings
Over time, this compound has been observed to increase the level of endogenous ROS in H1299 cells . This suggests that this compound may have long-term effects on cellular function, potentially influencing cell signaling pathways, gene expression, and cellular metabolism.
Dosage Effects in Animal Models
The effects of this compound vary with different dosages in animal models. It has been shown to effectively improve postoperative cognitive dysfunction (POCD) of mice after being accepted carotid artery exposure .
Metabolic Pathways
This compound is involved in several metabolic pathways. It can be generated from de novo synthesis, sphingomyelin hydrolysis, and/or the salvage pathway . Additionally, ceramide can be synthesized through the dephosphorylation of ceramide 1-phosphate (C1P) by ceramide 1-phosphate phosphatase (CPP) .
Transport and Distribution
This compound is an integral membrane protein of the endoplasmic reticulum . It utilizes fatty acyl CoAs of relatively defined chain lengths for N-acylation of the sphingoid long chain base .
Subcellular Localization
This compound, being an integral membrane protein of the endoplasmic reticulum, plays a crucial role in maintaining the structure of the plasma membrane and mediating numerous cell-signaling events .
Preparation Methods
Synthetic Routes and Reaction Conditions
C8-Ceramide can be synthesized through various chemical routes. One common method involves the acylation of sphingosine with octanoyl chloride in the presence of a base such as pyridine. The reaction typically occurs at room temperature and yields C8-Ceramide after purification .
Industrial Production Methods
Industrial production of C8-Ceramide often involves large-scale synthesis using similar acylation reactions. The process may include additional steps for purification and quality control to ensure the compound meets the required standards for research and therapeutic use .
Chemical Reactions Analysis
Types of Reactions
C8-Ceramide undergoes several types of chemical reactions, including:
Oxidation: C8-Ceramide can be oxidized to form ceramide-1-phosphate.
Reduction: Reduction of C8-Ceramide can yield dihydroceramide.
Substitution: Various substitution reactions can modify the functional groups on the ceramide molecule.
Common Reagents and Conditions
Oxidation: Common oxidizing agents include hydrogen peroxide and peracids.
Reduction: Reducing agents such as sodium borohydride are often used.
Substitution: Reagents like alkyl halides and nucleophiles are employed in substitution reactions.
Major Products Formed
Oxidation: Ceramide-1-phosphate
Reduction: Dihydroceramide
Substitution: Various substituted ceramides depending on the reagents used.
Comparison with Similar Compounds
C8-Ceramide is compared with other ceramide analogs such as:
C2-Ceramide: A shorter-chain analog that is less effective in mimicking natural ceramide functions.
C6-Ceramide: Another analog with a shorter fatty acid chain, often used in similar research applications.
Dihydroceramide: A reduced form of ceramide that lacks the double bond in the sphingosine backbone.
C8-Ceramide is unique due to its optimal chain length, which allows it to closely mimic the biological activities of natural ceramides while being more stable and easier to handle in laboratory settings .
Properties
IUPAC Name |
N-[(E,2S,3R)-1,3-dihydroxyoctadec-4-en-2-yl]octanamide | |
---|---|---|
Source | PubChem | |
URL | https://pubchem.ncbi.nlm.nih.gov | |
Description | Data deposited in or computed by PubChem | |
InChI |
InChI=1S/C26H51NO3/c1-3-5-7-9-10-11-12-13-14-15-16-18-19-21-25(29)24(23-28)27-26(30)22-20-17-8-6-4-2/h19,21,24-25,28-29H,3-18,20,22-23H2,1-2H3,(H,27,30)/b21-19+/t24-,25+/m0/s1 | |
Source | PubChem | |
URL | https://pubchem.ncbi.nlm.nih.gov | |
Description | Data deposited in or computed by PubChem | |
InChI Key |
APDLCSPGWPLYEQ-WRBRXSDHSA-N | |
Source | PubChem | |
URL | https://pubchem.ncbi.nlm.nih.gov | |
Description | Data deposited in or computed by PubChem | |
Canonical SMILES |
CCCCCCCCCCCCCC=CC(C(CO)NC(=O)CCCCCCC)O | |
Source | PubChem | |
URL | https://pubchem.ncbi.nlm.nih.gov | |
Description | Data deposited in or computed by PubChem | |
Isomeric SMILES |
CCCCCCCCCCCCC/C=C/[C@H]([C@H](CO)NC(=O)CCCCCCC)O | |
Source | PubChem | |
URL | https://pubchem.ncbi.nlm.nih.gov | |
Description | Data deposited in or computed by PubChem | |
Molecular Formula |
C26H51NO3 | |
Source | PubChem | |
URL | https://pubchem.ncbi.nlm.nih.gov | |
Description | Data deposited in or computed by PubChem | |
DSSTOX Substance ID |
DTXSID701318293 | |
Record name | C8-Ceramide | |
Source | EPA DSSTox | |
URL | https://comptox.epa.gov/dashboard/DTXSID701318293 | |
Description | DSSTox provides a high quality public chemistry resource for supporting improved predictive toxicology. | |
Molecular Weight |
425.7 g/mol | |
Source | PubChem | |
URL | https://pubchem.ncbi.nlm.nih.gov | |
Description | Data deposited in or computed by PubChem | |
CAS No. |
74713-59-0 | |
Record name | C8-Ceramide | |
Source | CAS Common Chemistry | |
URL | https://commonchemistry.cas.org/detail?cas_rn=74713-59-0 | |
Description | CAS Common Chemistry is an open community resource for accessing chemical information. Nearly 500,000 chemical substances from CAS REGISTRY cover areas of community interest, including common and frequently regulated chemicals, and those relevant to high school and undergraduate chemistry classes. This chemical information, curated by our expert scientists, is provided in alignment with our mission as a division of the American Chemical Society. | |
Explanation | The data from CAS Common Chemistry is provided under a CC-BY-NC 4.0 license, unless otherwise stated. | |
Record name | C8-Ceramide | |
Source | EPA DSSTox | |
URL | https://comptox.epa.gov/dashboard/DTXSID701318293 | |
Description | DSSTox provides a high quality public chemistry resource for supporting improved predictive toxicology. | |
Retrosynthesis Analysis
AI-Powered Synthesis Planning: Our tool employs the Template_relevance Pistachio, Template_relevance Bkms_metabolic, Template_relevance Pistachio_ringbreaker, Template_relevance Reaxys, Template_relevance Reaxys_biocatalysis model, leveraging a vast database of chemical reactions to predict feasible synthetic routes.
One-Step Synthesis Focus: Specifically designed for one-step synthesis, it provides concise and direct routes for your target compounds, streamlining the synthesis process.
Accurate Predictions: Utilizing the extensive PISTACHIO, BKMS_METABOLIC, PISTACHIO_RINGBREAKER, REAXYS, REAXYS_BIOCATALYSIS database, our tool offers high-accuracy predictions, reflecting the latest in chemical research and data.
Strategy Settings
Precursor scoring | Relevance Heuristic |
---|---|
Min. plausibility | 0.01 |
Model | Template_relevance |
Template Set | Pistachio/Bkms_metabolic/Pistachio_ringbreaker/Reaxys/Reaxys_biocatalysis |
Top-N result to add to graph | 6 |
Feasible Synthetic Routes
Disclaimer and Information on In-Vitro Research Products
Please be aware that all articles and product information presented on BenchChem are intended solely for informational purposes. The products available for purchase on BenchChem are specifically designed for in-vitro studies, which are conducted outside of living organisms. In-vitro studies, derived from the Latin term "in glass," involve experiments performed in controlled laboratory settings using cells or tissues. It is important to note that these products are not categorized as medicines or drugs, and they have not received approval from the FDA for the prevention, treatment, or cure of any medical condition, ailment, or disease. We must emphasize that any form of bodily introduction of these products into humans or animals is strictly prohibited by law. It is essential to adhere to these guidelines to ensure compliance with legal and ethical standards in research and experimentation.