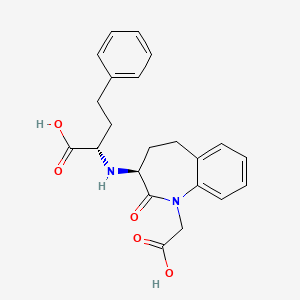
Benazeprilat
Overview
Description
Benazeprilat is the active metabolite of the prodrug benazepril. It is a non-sulfhydryl angiotensin-converting enzyme (ACE) inhibitor used primarily in the treatment of hypertension, heart failure, and chronic renal failure. This compound works by inhibiting the conversion of angiotensin I to angiotensin II, a potent vasoconstrictor, thereby reducing blood pressure and improving cardiac function .
Mechanism of Action
Target of Action
Benazeprilat, the active metabolite of Benazepril, primarily targets the angiotensin-converting enzyme (ACE) . ACE plays a crucial role in the Renin-Angiotensin-Aldosterone System (RAAS), which regulates blood pressure and fluid balance in the body .
Pharmacokinetics
Benazepril, the prodrug, is converted into its active form, this compound, primarily in the liver . The absorption, bioactivation, distribution, and elimination of benazepril and this compound have been evaluated in healthy subjects, hypertensive patients, and patients with characteristics known to alter the pharmacokinetic disposition of ACE inhibitors, such as renal impairment, hepatic impairment, and advanced age .
Result of Action
The molecular and cellular effects of this compound’s action primarily involve the disruption of the RAAS pathway . This results in vasodilation (due to decreased angiotensin II) and decreased fluid volume (due to reduced aldosterone secretion), leading to a significant reduction in blood pressure . This makes this compound effective in the treatment of conditions like hypertension and congestive heart failure .
Action Environment
The action, efficacy, and stability of this compound can be influenced by various environmental factors. For instance, the conversion of Benazepril to this compound can be slowed in the presence of severe hepatic impairment . Additionally, severe renal impairment can reduce this compound elimination and require dosage reduction . Age can also influence the disposition of this compound, with clearance slightly reduced in elderly patients .
Biochemical Analysis
Biochemical Properties
Benazeprilat exerts its effects by inhibiting the angiotensin-converting enzyme (ACE), a critical enzyme in the renin-angiotensin-aldosterone system . This system plays a key role in regulating blood pressure and fluid balance. By inhibiting ACE, this compound prevents the conversion of angiotensin I to the potent vasoconstrictor, angiotensin II . This leads to vasodilation, reduced blood volume, and ultimately, lower blood pressure .
Cellular Effects
This compound’s primary cellular effect is the reduction of angiotensin II levels. This decrease in angiotensin II leads to a reduction in vasoconstriction and a decrease in the release of aldosterone, a hormone that promotes sodium and water retention . These effects collectively result in a decrease in blood pressure .
Molecular Mechanism
The molecular mechanism of this compound involves the competitive inhibition of ACE. By binding to ACE, this compound prevents the enzyme from converting angiotensin I to angiotensin II . This inhibition leads to decreased levels of angiotensin II, resulting in vasodilation and decreased blood volume .
Temporal Effects in Laboratory Settings
In laboratory settings, the effects of this compound are observed over time. Following administration, peak plasma levels of this compound are observed between 1 and 6 hours . The elimination of this compound from plasma is biphasic, with mean initial and terminal half-lives of 3.0 and 17.3 hours, respectively .
Dosage Effects in Animal Models
In animal models, the effects of this compound have been observed to vary with dosage . While specific dosage effects can depend on the model used, it is generally observed that higher dosages lead to greater reductions in blood pressure .
Metabolic Pathways
This compound is involved in the metabolic pathway of benazepril . Benazepril is metabolized in the liver, where it undergoes enzymatic hydrolysis to form this compound . This process involves the cleavage of an ester group, transforming the prodrug benazepril into its active form .
Transport and Distribution
After oral administration, benazepril is absorbed and transformed into this compound in the liver . The distribution volume of this compound is approximately 8.7 L . It is primarily excreted in the urine, with trace amounts of benazepril and about 20% as this compound .
Subcellular Localization
The subcellular localization of this compound is not explicitly defined in the literature. Given its role as an ACE inhibitor, it is likely to be found wherever ACE is present within the cell. ACE is typically found in the endothelial cells of the blood vessels, renal tubular epithelium, and various other tissues .
Preparation Methods
Synthetic Routes and Reaction Conditions: The synthesis of benazeprilat typically involves the hydrolysis of benazepril. Benazepril is first synthesized through a multi-step process that includes the condensation of a benzazepine derivative with a phenylbutanoic acid derivative. The final step involves the hydrolysis of benazepril to this compound using sodium hydroxide under controlled conditions .
Industrial Production Methods: In industrial settings, this compound is produced by hydrolyzing benazepril hydrochloride. The process involves dissolving benazepril hydrochloride in a suitable solvent, followed by the addition of a base such as sodium hydroxide to induce hydrolysis. The reaction mixture is then purified through crystallization or chromatography to obtain this compound in high purity .
Chemical Reactions Analysis
Types of Reactions: Benazeprilat undergoes several types of chemical reactions, including hydrolysis, oxidation, and substitution reactions.
Common Reagents and Conditions:
Hydrolysis: Sodium hydroxide is commonly used to hydrolyze benazepril to this compound.
Oxidation: this compound can be oxidized using oxidizing agents such as hydrogen peroxide under controlled conditions.
Substitution: this compound can undergo substitution reactions with various electrophiles in the presence of suitable catalysts.
Major Products: The primary product of the hydrolysis of benazepril is this compound. Oxidation and substitution reactions can yield various derivatives of this compound, depending on the reagents and conditions used .
Scientific Research Applications
Benazeprilat has a wide range of scientific research applications:
Chemistry: It is used as a reference compound in the study of ACE inhibitors and their mechanisms of action.
Biology: this compound is used in research to understand the role of the renin-angiotensin-aldosterone system in various physiological and pathological conditions.
Medicine: It is extensively studied for its therapeutic effects in hypertension, heart failure, and renal diseases.
Comparison with Similar Compounds
Properties
IUPAC Name |
(2S)-2-[[(3S)-1-(carboxymethyl)-2-oxo-4,5-dihydro-3H-1-benzazepin-3-yl]amino]-4-phenylbutanoic acid | |
---|---|---|
Source | PubChem | |
URL | https://pubchem.ncbi.nlm.nih.gov | |
Description | Data deposited in or computed by PubChem | |
InChI |
InChI=1S/C22H24N2O5/c25-20(26)14-24-19-9-5-4-8-16(19)11-13-17(21(24)27)23-18(22(28)29)12-10-15-6-2-1-3-7-15/h1-9,17-18,23H,10-14H2,(H,25,26)(H,28,29)/t17-,18-/m0/s1 | |
Source | PubChem | |
URL | https://pubchem.ncbi.nlm.nih.gov | |
Description | Data deposited in or computed by PubChem | |
InChI Key |
MADRIHWFJGRSBP-ROUUACIJSA-N | |
Source | PubChem | |
URL | https://pubchem.ncbi.nlm.nih.gov | |
Description | Data deposited in or computed by PubChem | |
Canonical SMILES |
C1CC2=CC=CC=C2N(C(=O)C1NC(CCC3=CC=CC=C3)C(=O)O)CC(=O)O | |
Source | PubChem | |
URL | https://pubchem.ncbi.nlm.nih.gov | |
Description | Data deposited in or computed by PubChem | |
Isomeric SMILES |
C1CC2=CC=CC=C2N(C(=O)[C@H]1N[C@@H](CCC3=CC=CC=C3)C(=O)O)CC(=O)O | |
Source | PubChem | |
URL | https://pubchem.ncbi.nlm.nih.gov | |
Description | Data deposited in or computed by PubChem | |
Molecular Formula |
C22H24N2O5 | |
Source | PubChem | |
URL | https://pubchem.ncbi.nlm.nih.gov | |
Description | Data deposited in or computed by PubChem | |
DSSTOX Substance ID |
DTXSID501024701 | |
Record name | Benazeprilat | |
Source | EPA DSSTox | |
URL | https://comptox.epa.gov/dashboard/DTXSID501024701 | |
Description | DSSTox provides a high quality public chemistry resource for supporting improved predictive toxicology. | |
Molecular Weight |
396.4 g/mol | |
Source | PubChem | |
URL | https://pubchem.ncbi.nlm.nih.gov | |
Description | Data deposited in or computed by PubChem | |
CAS No. |
86541-78-8 | |
Record name | Benazeprilat | |
Source | CAS Common Chemistry | |
URL | https://commonchemistry.cas.org/detail?cas_rn=86541-78-8 | |
Description | CAS Common Chemistry is an open community resource for accessing chemical information. Nearly 500,000 chemical substances from CAS REGISTRY cover areas of community interest, including common and frequently regulated chemicals, and those relevant to high school and undergraduate chemistry classes. This chemical information, curated by our expert scientists, is provided in alignment with our mission as a division of the American Chemical Society. | |
Explanation | The data from CAS Common Chemistry is provided under a CC-BY-NC 4.0 license, unless otherwise stated. | |
Record name | Benazeprilat [USAN:INN] | |
Source | ChemIDplus | |
URL | https://pubchem.ncbi.nlm.nih.gov/substance/?source=chemidplus&sourceid=0086541788 | |
Description | ChemIDplus is a free, web search system that provides access to the structure and nomenclature authority files used for the identification of chemical substances cited in National Library of Medicine (NLM) databases, including the TOXNET system. | |
Record name | Benazeprilat | |
Source | DrugBank | |
URL | https://www.drugbank.ca/drugs/DB14125 | |
Description | The DrugBank database is a unique bioinformatics and cheminformatics resource that combines detailed drug (i.e. chemical, pharmacological and pharmaceutical) data with comprehensive drug target (i.e. sequence, structure, and pathway) information. | |
Explanation | Creative Common's Attribution-NonCommercial 4.0 International License (http://creativecommons.org/licenses/by-nc/4.0/legalcode) | |
Record name | Benazeprilat | |
Source | EPA DSSTox | |
URL | https://comptox.epa.gov/dashboard/DTXSID501024701 | |
Description | DSSTox provides a high quality public chemistry resource for supporting improved predictive toxicology. | |
Record name | BENAZEPRILAT | |
Source | FDA Global Substance Registration System (GSRS) | |
URL | https://gsrs.ncats.nih.gov/ginas/app/beta/substances/JRM708L703 | |
Description | The FDA Global Substance Registration System (GSRS) enables the efficient and accurate exchange of information on what substances are in regulated products. Instead of relying on names, which vary across regulatory domains, countries, and regions, the GSRS knowledge base makes it possible for substances to be defined by standardized, scientific descriptions. | |
Explanation | Unless otherwise noted, the contents of the FDA website (www.fda.gov), both text and graphics, are not copyrighted. They are in the public domain and may be republished, reprinted and otherwise used freely by anyone without the need to obtain permission from FDA. Credit to the U.S. Food and Drug Administration as the source is appreciated but not required. | |
Retrosynthesis Analysis
AI-Powered Synthesis Planning: Our tool employs the Template_relevance Pistachio, Template_relevance Bkms_metabolic, Template_relevance Pistachio_ringbreaker, Template_relevance Reaxys, Template_relevance Reaxys_biocatalysis model, leveraging a vast database of chemical reactions to predict feasible synthetic routes.
One-Step Synthesis Focus: Specifically designed for one-step synthesis, it provides concise and direct routes for your target compounds, streamlining the synthesis process.
Accurate Predictions: Utilizing the extensive PISTACHIO, BKMS_METABOLIC, PISTACHIO_RINGBREAKER, REAXYS, REAXYS_BIOCATALYSIS database, our tool offers high-accuracy predictions, reflecting the latest in chemical research and data.
Strategy Settings
Precursor scoring | Relevance Heuristic |
---|---|
Min. plausibility | 0.01 |
Model | Template_relevance |
Template Set | Pistachio/Bkms_metabolic/Pistachio_ringbreaker/Reaxys/Reaxys_biocatalysis |
Top-N result to add to graph | 6 |
Feasible Synthetic Routes
Q1: How does benazeprilat interact with its target and what are the downstream effects of this interaction?
A1: this compound is the active metabolite of benazepril, functioning as a potent angiotensin-converting enzyme (ACE) inhibitor []. It achieves this by competitively binding to and inhibiting ACE [, ]. This binding prevents the conversion of angiotensin I to angiotensin II [, , ]. As a result, the potent vasoconstrictive actions of angiotensin II are suppressed, leading to vasodilation [, ]. Additionally, this compound diminishes angiotensin II-induced aldosterone secretion by the adrenal cortex, promoting sodium excretion and subsequently increasing water outflow [, , ].
Q2: What is the molecular formula and weight of this compound?
A2: The molecular formula of this compound is C24H28N2O5. Its molecular weight is 424.5 g/mol.
Q3: Is there any information on the performance and applications of this compound under various conditions?
A3: While specific details regarding material compatibility and stability under various conditions are limited within the provided research, studies highlight the importance of appropriate formulation strategies to enhance its stability, solubility, and bioavailability []. Further investigation is necessary to fully elucidate its performance and applications under diverse environmental conditions.
Q4: Does this compound possess any catalytic properties?
A4: this compound is not known to exhibit catalytic properties. Its primary mechanism of action revolves around the inhibition of ACE, rather than catalyzing any specific chemical reaction.
Q5: How do modifications to the structure of this compound affect its activity, potency, and selectivity?
A6: Research indicates that the SS configuration of this compound exhibits the most potent ACE inhibitory activity []. While precise details regarding the impact of specific structural modifications are limited in the provided research, exploring these alterations could unveil valuable insights into optimizing this compound's therapeutic profile.
Q6: Is there any information regarding SHE regulations and compliance for this compound?
A6: The provided scientific research does not explicitly discuss SHE regulations related to this compound. Adherence to relevant safety guidelines and regulations is paramount throughout all stages of its development, manufacturing, and distribution.
Q7: What is known about the ADME profile of this compound and its in vivo activity and efficacy?
A9: Benazepril, the prodrug of this compound, undergoes rapid absorption and hepatic metabolism to its active form, this compound [, , , , , , , , ]. this compound exhibits a long duration of action due to its high affinity binding to ACE [, , , ]. The elimination of this compound occurs primarily through biliary excretion [, , ], with a minor contribution from renal excretion [, , ]. It demonstrates potent and long-lasting ACE inhibition in various animal models [, , , , ], contributing to its antihypertensive effects [, , , , , ].
Q8: What are the findings from cell-based assays, animal models, and clinical trials regarding the efficacy of this compound?
A10: this compound effectively inhibits ACE activity in both in vitro and in vivo settings. In isolated vascular preparations, it demonstrates potent inhibition of angiotensin I-induced contractions []. Animal studies highlight its ability to lower blood pressure [, , , , , ], improve left ventricular function [, ], and protect against end-organ damage [, ]. While human clinical trials are not explicitly discussed in the provided research, the collective findings from these studies support its potential as a therapeutic agent.
Disclaimer and Information on In-Vitro Research Products
Please be aware that all articles and product information presented on BenchChem are intended solely for informational purposes. The products available for purchase on BenchChem are specifically designed for in-vitro studies, which are conducted outside of living organisms. In-vitro studies, derived from the Latin term "in glass," involve experiments performed in controlled laboratory settings using cells or tissues. It is important to note that these products are not categorized as medicines or drugs, and they have not received approval from the FDA for the prevention, treatment, or cure of any medical condition, ailment, or disease. We must emphasize that any form of bodily introduction of these products into humans or animals is strictly prohibited by law. It is essential to adhere to these guidelines to ensure compliance with legal and ethical standards in research and experimentation.