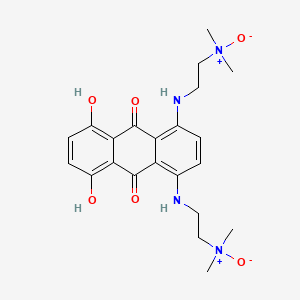
Banoxantrone
Overview
Description
Banoxantrone is a highly selective bioreductive drug that is activated in, and is preferentially toxic to, hypoxic cells in tumors. It has shown efficacy in combination with radiation and chemotherapy, significantly delaying tumor growth compared to either treatment alone .
Preparation Methods
Synthetic Routes and Reaction Conditions: Banoxantrone is synthesized through a series of chemical reactions involving the formation of its core structure, followed by functionalization to achieve the final compound. The synthesis typically involves the use of organic solvents, catalysts, and specific reaction conditions to ensure high yield and purity .
Industrial Production Methods: Industrial production of this compound involves scaling up the laboratory synthesis process. This includes optimizing reaction conditions, using large-scale reactors, and implementing purification techniques such as crystallization and chromatography to obtain the final product in bulk quantities .
Chemical Reactions Analysis
Types of Reactions: Banoxantrone undergoes various chemical reactions, including:
Oxidation: Conversion to its active form in hypoxic conditions.
Reduction: Bioreduction by tissue cytochrome P450 to its cytotoxic form.
Common Reagents and Conditions:
Oxidation: Requires hypoxic conditions and the presence of specific enzymes.
Reduction: Involves tissue cytochrome P450 and other reductases.
Major Products Formed: The major product formed from the bioreduction of this compound is its cytotoxic form, which intercalates DNA and inhibits topoisomerase II .
Scientific Research Applications
Banoxantrone has a wide range of applications in scientific research, including:
Chemistry: Used as a model compound to study bioreductive activation and hypoxia-targeted therapies.
Medicine: Explored as a treatment for various forms of cancer, particularly those with hypoxic tumor regions.
Industry: Utilized in the development of new cancer therapies and drug delivery systems.
Mechanism of Action
Banoxantrone is preferentially and irreversibly converted to its cytotoxic form in hypoxic tumor cells. This conversion is facilitated by tissue cytochrome P450 and other reductases. The cytotoxic form intercalates DNA and inhibits topoisomerase II, leading to cell death. This mechanism targets hypoxic tumor cells while sparing oxygenated cells .
Comparison with Similar Compounds
Mitoxantrone: Another DNA intercalator and topoisomerase II inhibitor used in cancer therapy.
Cisplatin: A platinum-based chemotherapy drug that forms DNA crosslinks.
Thiotepa: An alkylating agent used in chemotherapy.
Uniqueness of Banoxantrone: this compound’s uniqueness lies in its selective activation in hypoxic conditions, making it particularly effective against hypoxic tumor cells. This selective toxicity reduces damage to healthy, oxygenated tissues and enhances the efficacy of combination therapies .
Properties
Key on ui mechanism of action |
Banoxantrone (formally known as AQ4N) is preferentially and irreversibly converted to AQ4, its cytotoxic form, in hypoxic tumour cells where it remains localised. When the surrounding oxygenated cells are killed by radiotherapy or chemotherapy bringing these AQ4-containing quiescent cells closer to the oxygen source, they become reoxygenated, attempt to resume replication and, in this state, are killed by AQ4 through potent DNA intercalation and topoisomerase II inhibition. |
---|---|
CAS No. |
136470-65-0 |
Molecular Formula |
C22H28N4O6 |
Molecular Weight |
444.5 g/mol |
IUPAC Name |
2-[[4-[2-[dimethyl(oxido)azaniumyl]ethylamino]-5,8-dihydroxy-9,10-dioxoanthracen-1-yl]amino]-N,N-dimethylethanamine oxide |
InChI |
InChI=1S/C22H28N4O6/c1-25(2,31)11-9-23-13-5-6-14(24-10-12-26(3,4)32)18-17(13)21(29)19-15(27)7-8-16(28)20(19)22(18)30/h5-8,23-24,27-28H,9-12H2,1-4H3 |
InChI Key |
YZBAXVICWUUHGG-UHFFFAOYSA-N |
SMILES |
C[N+](C)(CCNC1=C2C(=C(C=C1)NCC[N+](C)(C)[O-])C(=O)C3=C(C=CC(=C3C2=O)O)O)[O-] |
Canonical SMILES |
C[N+](C)(CCNC1=C2C(=C(C=C1)NCC[N+](C)(C)[O-])C(=O)C3=C(C=CC(=C3C2=O)O)O)[O-] |
Appearance |
Solid powder |
Key on ui other cas no. |
136470-65-0 |
Purity |
>98% (or refer to the Certificate of Analysis) |
shelf_life |
>5 years if stored properly |
solubility |
Soluble in DMSO, not in water |
storage |
Dry, dark and at 0 - 4 C for short term (days to weeks) or -20 C for long term (months to years). |
Synonyms |
1,4-bis-((2-(dimethylamino)ethyl)amino)-5,8-dihydroxyanthracene-9,10-dione 1,4-bis-((2-(dimethylamino-N-oxide)ethyl)amino)-5,8-dihydroxyanthracene-9,10-dione AQ4N banoxantrone banoxantrone dihydrochloride |
Origin of Product |
United States |
Retrosynthesis Analysis
AI-Powered Synthesis Planning: Our tool employs the Template_relevance Pistachio, Template_relevance Bkms_metabolic, Template_relevance Pistachio_ringbreaker, Template_relevance Reaxys, Template_relevance Reaxys_biocatalysis model, leveraging a vast database of chemical reactions to predict feasible synthetic routes.
One-Step Synthesis Focus: Specifically designed for one-step synthesis, it provides concise and direct routes for your target compounds, streamlining the synthesis process.
Accurate Predictions: Utilizing the extensive PISTACHIO, BKMS_METABOLIC, PISTACHIO_RINGBREAKER, REAXYS, REAXYS_BIOCATALYSIS database, our tool offers high-accuracy predictions, reflecting the latest in chemical research and data.
Strategy Settings
Precursor scoring | Relevance Heuristic |
---|---|
Min. plausibility | 0.01 |
Model | Template_relevance |
Template Set | Pistachio/Bkms_metabolic/Pistachio_ringbreaker/Reaxys/Reaxys_biocatalysis |
Top-N result to add to graph | 6 |
Feasible Synthetic Routes
Disclaimer and Information on In-Vitro Research Products
Please be aware that all articles and product information presented on BenchChem are intended solely for informational purposes. The products available for purchase on BenchChem are specifically designed for in-vitro studies, which are conducted outside of living organisms. In-vitro studies, derived from the Latin term "in glass," involve experiments performed in controlled laboratory settings using cells or tissues. It is important to note that these products are not categorized as medicines or drugs, and they have not received approval from the FDA for the prevention, treatment, or cure of any medical condition, ailment, or disease. We must emphasize that any form of bodily introduction of these products into humans or animals is strictly prohibited by law. It is essential to adhere to these guidelines to ensure compliance with legal and ethical standards in research and experimentation.