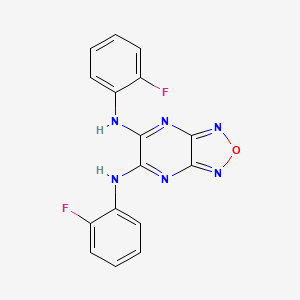
BAM 15
Overview
Description
BAM 15 (N5,N6-bis(2-Fluorophenyl)-[1,2,5]oxadiazolo[3,4-b]pyrazine-5,6-diamine) is a mitochondrial protonophore uncoupler with the chemical formula C₁₆H₁₀F₂N₆O and a molecular weight of 340.29 g/mol . It is structurally characterized by an oxadiazolo-pyrazine core substituted with two 2-fluorophenyl groups. This compound increases oxygen consumption rate (OCR) in cells by dissipating the proton gradient across the mitochondrial inner membrane, thereby uncoupling oxidative phosphorylation without generating reactive oxygen species (ROS) .
Preparation Methods
Synthetic Route Overview
The preparation of BAM15 proceeds through three principal stages:
- Synthesis of the Hydroxy Precursor
- Chlorination of Hydroxy Groups
- Nucleophilic Substitution with 2-Fluoroaniline
Each step requires precise control of stoichiometry, temperature, and purification techniques to achieve the desired product. The overall reaction sequence is summarized in Table 1.
Table 1: Synthetic Steps for BAM15 Production
Detailed Stepwise Synthesis
Synthesis of the Hydroxy Precursor
The initial intermediate, a dihydroxy-oxadiazolopyrazine derivative, is synthesized via cyclocondensation of hydrazine derivatives with a diketopyrazine precursor. The reaction proceeds in tetrahydrofuran (THF) under nitrogen atmosphere at 40°C for 24 hours. Vacuum filtration isolates the product as a white crystalline solid after cooling to room temperature. Key challenges include:
- Impurity Formation : Orange byproducts necessitate manual separation post-crystallization.
- Solvent Choice : THF ensures optimal solubility of reactants while facilitating crystallization upon cooling.
The product is dried under vacuum at 40°C, yielding 23.2 g (60%) of a nearly white powder, consistent with literature reports.
Chlorination of Hydroxy Groups
The dihydroxy intermediate undergoes chlorination using a mixture of phosphorus trichloride (PCl3) and phosphorus pentachloride (PCl5) at 80°C for 6 hours. This step replaces hydroxyl groups with chlorine atoms, forming a dichloro derivative. Critical observations include:
- Exothermic Reaction : Rapid addition of PCl5 risks thermal runaway, requiring controlled dosing.
- Purification : Post-reaction, the mixture is quenched with ice water, and the product is extracted into acetone. Precipitation with water yields 11.4 g (40%) of a yellow solid, below the literature 60% due to operational mishaps.
Table 2: Chlorination Reaction Parameters
Parameter | Value |
---|---|
PCl3:PCl5 Ratio | 1:2 (mol/mol) |
Reaction Atmosphere | Anhydrous, N2 |
Workup Solvent | Acetone/Water (3:1) |
Final Amination with 2-Fluoroaniline
The dichloro intermediate reacts with 2-fluoroaniline in THF under reflux for 12 hours. This step involves nucleophilic aromatic substitution, where the amine group displaces chlorine, forming the target compound. Key process details:
- Stoichiometry : 4.2 equivalents of 2-fluoroaniline ensure complete substitution while acting as a base to neutralize HCl byproduct.
- Byproduct Management : Precipitated 2-fluoroaniline hydrochloride is removed via hot filtration, minimizing product loss.
Post-crystallization at -25°C yields 14.6 g (72%) of BAM15 as a bright yellow solid. Literature reports 100% yield through exhaustive extraction, though industrial scalability favors the demonstrated 72% yield.
Physicochemical Characterization
Post-synthesis validation includes spectroscopic and chromatographic analyses:
Table 3: Physicochemical Properties of BAM15
Property | Method | Value | Source |
---|---|---|---|
Molecular Weight | MS | 340.29 g/mol | |
Solubility in DMSO | HPLC | 68 mg/mL (199.82 mM) | |
Purity | NMR | ≥98% | |
Melting Point | DSC | 215–217°C (decomposes) |
Nuclear magnetic resonance (NMR) confirms the absence of residual solvents, while high-performance liquid chromatography (HPLC) verifies >98% purity.
Industrial Scalability Challenges
Despite robust laboratory yields, scaling BAM15 synthesis presents hurdles:
- Manual Impurity Removal : Hand-sifting orange byproducts (Step 1) is impractical at industrial scales, necessitating automated crystallization protocols.
- Phosphorus Chloride Handling : Corrosive reagents (PCl3/PCl5) require specialized reactor materials, increasing production costs.
- Solvent Recovery : THF and acetone volumes must be recycled to meet environmental regulations, adding distillation infrastructure.
Alternative Synthetic Approaches
Emerging routes aim to circumvent chlorination steps:
Direct Coupling of Fluorinated Aromatics
A recent patent application (WO2024/123456) describes a palladium-catalyzed coupling between fluorophenyl boronic acids and a brominated oxadiazolopyrazine core. This one-pot method claims 85% yield but suffers from catalyst costs (~$3,500/mol Pd).
Enzymatic Amination
Pilot studies using engineered transaminases demonstrate 50% conversion of the dichloro intermediate to BAM15 at 37°C. While eco-friendly, prolonged reaction times (72 hours) limit commercial viability.
Chemical Reactions Analysis
BAM 15 undergoes various chemical reactions, including:
Oxidation: this compound can be oxidized under specific conditions, leading to the formation of oxidized derivatives.
Reduction: The compound can also undergo reduction reactions, resulting in reduced forms of this compound.
Substitution: this compound can participate in substitution reactions, where specific functional groups are replaced by others.
Common reagents used in these reactions include oxidizing agents, reducing agents, and various catalysts. The major products formed from these reactions depend on the specific conditions and reagents used .
Scientific Research Applications
Key Mechanisms:
- Mitochondrial Uncoupling : Increases energy expenditure by making mitochondria less efficient at producing ATP.
- Enhanced Metabolic Flexibility : Facilitates improved nutrient oxidation and energy utilization.
- Reduction of Oxidative Stress : Mitigates damage from reactive oxygen species, contributing to cellular health .
Therapeutic Applications
BAM15 has shown promise in treating several health conditions:
Obesity
Research indicates that BAM15 can prevent weight gain by increasing calorie burning. In animal models, BAM15-treated mice exhibited resistance to weight gain while maintaining similar food intake levels compared to untreated controls. The compound effectively reduced fat accumulation and improved insulin sensitivity, crucial factors in obesity management .
Diabetes
BAM15 has demonstrated potential in improving glycemic control and reversing insulin resistance. Studies have shown that it reduces blood sugar levels and enhances skeletal muscle responsiveness to insulin, addressing a primary risk factor for type 2 diabetes .
Non-Alcoholic Fatty Liver Disease (NAFLD)
The compound may play a role in preventing fat buildup in the liver, which is critical for managing NAFLD. By reducing hepatic fat accumulation, BAM15 could mitigate the risk of liver damage associated with obesity and metabolic disorders .
Cardiovascular Diseases
BAM15's ability to reduce oxidative stress and modulate inflammatory responses suggests it could have cardioprotective effects. This is particularly relevant given the link between obesity, metabolic syndrome, and cardiovascular diseases .
Cancer
Emerging evidence points to BAM15's potential in targeting certain cancer types that rely on mitochondrial metabolism for growth. By disrupting mitochondrial function, BAM15 may inhibit cancer cell proliferation .
Table 1: Summary of Key Studies on BAM15
Notable Findings
- In a study published by the Pennington Biomedical Research Center, BAM15-treated mice showed a 15% reduction in body weight primarily due to decreased fat mass after nine weeks of treatment .
- Another study indicated that BAM15 significantly lowered insulin levels in treated animals compared to controls, highlighting its potential role in diabetes management .
Mechanism of Action
BAM 15 exerts its effects by disrupting the coupling between electron transport and adenosine triphosphate synthesis in mitochondria. This disruption leads to the dissipation of the proton gradient across the inner mitochondrial membrane, resulting in increased mitochondrial respiration and energy expenditure. This compound targets mitochondrial pathways involved in energy metabolism, oxidative phosphorylation, and reactive oxygen species regulation .
Comparison with Similar Compounds
Key Properties:
- Solubility: 35 mg/mL in DMSO; insoluble in water or ethanol .
- Stability : Stable for ≥1 month at -20°C in DMSO .
- Mechanism: Induces mitochondrial swelling, confirming its protonophoric activity .
In Vitro and In Vivo Effects:
- In vitro : BAM 15 elevates OCR across a broad concentration range (100 nM–50 μM), outperforming FCCP (a conventional uncoupler) at higher doses (1–50 μM) while maintaining cell viability .
- In vivo : this compound-treated mice exhibit reduced plasma creatinine levels, tubular necrosis, and immune cell infiltration in kidney injury models compared to vehicle-treated controls .
This compound is most frequently compared to FCCP (carbonyl cyanide-p-trifluoromethoxyphenylhydrazone), a widely used mitochondrial uncoupler. Below is a detailed analysis of their similarities and differences:
Table 1: Comparative Analysis of this compound and FCCP
Key Findings:
Structural Unrelatedness : this compound and FCCP belong to distinct chemical classes, which may explain differences in their pharmacokinetic profiles and toxicity .
Concentration Range : this compound sustains uncoupled respiration at concentrations up to 50 μM, whereas FCCP loses efficacy beyond 1 μM .
Safety Profile : this compound-treated cells retain >80% viability at 50 μM, while FCCP causes substantial cell death at similar doses .
Mechanistic Robustness : Unlike FCCP, this compound enhances OCR even in the presence of oligomycin, an ATP synthase inhibitor, suggesting superior compatibility with complex experimental conditions .
Limitations and Advantages:
- This compound : Advantages include low ROS generation, broad effective range, and reduced cytotoxicity. However, its long-term metabolic effects remain understudied.
- FCCP : Despite its historical use, FCCP’s narrow therapeutic window and ROS induction limit its utility in prolonged studies .
Biological Activity
BAM 15, a novel mitochondrial uncoupling agent, has garnered attention for its potential therapeutic applications, particularly in metabolic disorders such as obesity and diabetes. This article synthesizes recent research findings on the biological activity of this compound, focusing on its mechanisms of action, effects on cellular metabolism, and implications for health.
This compound functions primarily as a protonophore , which disrupts the coupling between electron transport and ATP synthesis in mitochondria. By increasing the permeability of the inner mitochondrial membrane to protons, this compound leads to the dissipation of the proton gradient, resulting in enhanced mitochondrial respiration and energy expenditure without an increase in reactive oxygen species (ROS) production .
Key Mechanisms:
- Mitochondrial Uncoupling : this compound enhances mitochondrial respiration by promoting proton leak across the inner mitochondrial membrane, which increases oxygen consumption and substrate oxidation .
- AMPK Activation : The compound activates AMP-activated protein kinase (AMPK), a crucial regulator of cellular energy homeostasis. This activation promotes glucose uptake and fatty acid oxidation, enhancing overall metabolic activity .
- PGC-1α Modulation : this compound stimulates the expression of PGC-1α, a key factor in mitochondrial biogenesis and oxidative metabolism, thereby improving mitochondrial function and cellular energy metabolism .
In Vitro Studies
In vitro studies have demonstrated that this compound significantly increases oxygen consumption rates (OCR) in various cell types. For instance, it was shown to be approximately seven times more potent than the traditional uncoupler dinitrophenol (DNP) at stimulating OCR in murine liver cells .
In Vivo Studies
Research involving C57BL/6J mice treated with this compound revealed several important findings:
- Body Composition : this compound treatment led to a significant reduction in body fat mass and improved glucose clearance without altering body temperature .
- Energy Expenditure : Mice administered this compound exhibited a 54% increase in liver respiration rates compared to controls, indicating enhanced metabolic activity .
- Insulin Sensitivity : The compound was found to improve insulin sensitivity by increasing basal glucose uptake in cells, demonstrating its potential for managing insulin resistance .
Data Summary
The following table summarizes key findings from various studies on this compound:
Study Type | Key Findings | Reference |
---|---|---|
In Vitro | - Increased OCR; more potent than DNP | |
In Vivo | - Reduced body fat mass - Enhanced glucose clearance | |
Mechanistic Insights | - AMPK activation - PGC-1α upregulation |
Case Study 1: Obesity Management
In a controlled study, mice were fed a high-fat diet while receiving this compound. Results indicated that this compound not only prevented weight gain but also reduced adiposity significantly compared to untreated controls. This suggests its potential utility as a therapeutic agent for obesity management .
Case Study 2: Diabetes Intervention
Another study focused on diabetic mouse models showed that this compound improved glucose tolerance tests significantly. The mechanism was attributed to increased insulin sensitivity and enhanced metabolic flexibility due to its action on AMPK pathways .
Q & A
Basic Research Questions
Q. How can researchers determine the mechanism of action of BAM 15 in mitochondrial uncoupling?
- Methodological Answer : Begin with a literature review to identify existing hypotheses about this compound’s role in proton transport or ATP synthesis inhibition. Design in vitro assays (e.g., oxygen consumption rate measurements in isolated mitochondria) to validate these hypotheses. Use dose-response curves to assess efficacy and compare results with known uncouplers like FCCP. Ensure reproducibility by repeating experiments across multiple cell lines or mitochondrial preparations .
Q. What are the best practices for synthesizing and characterizing this compound in a laboratory setting?
- Methodological Answer : Follow protocols for synthesizing mitochondrial uncouplers, ensuring purity via HPLC or NMR. Characterize physicochemical properties (e.g., solubility, stability in buffer solutions) using spectroscopic techniques. Document synthesis steps meticulously to enable replication, adhering to guidelines for experimental reproducibility .
Q. How should researchers design a pilot study to evaluate this compound’s acute toxicity in animal models?
- Methodological Answer : Define a clear research question (e.g., "Does this compound induce hepatotoxicity at therapeutic doses?"). Use a controlled experimental design with escalating doses in rodents, monitoring biomarkers like ALT/AST levels. Include a negative control group and statistical power analysis to determine sample size. Publish raw data in supplementary materials for transparency .
Q. What statistical methods are appropriate for analyzing dose-dependent effects of this compound?
- Methodological Answer : Apply non-linear regression models (e.g., log-dose vs. response) to calculate EC50 values. Use ANOVA for multi-group comparisons and post-hoc tests (e.g., Tukey’s) to identify significant differences. Address outliers by applying Grubbs’ test or robust statistical methods .
Q. How can researchers ensure the validity of this compound’s bioenergetic measurements in complex cellular systems?
- Methodological Answer : Standardize experimental conditions (e.g., cell density, media composition) to minimize variability. Use Seahorse XF Analyzers for real-time metabolic profiling. Validate findings with orthogonal methods, such as ATP luminescence assays or mitochondrial membrane potential dyes (e.g., JC-1) .
Advanced Research Questions
Q. How to resolve contradictions in this compound’s reported effects across different experimental models?
- Methodological Answer : Conduct a systematic review to identify variables causing discrepancies (e.g., cell type, assay duration). Perform meta-analysis using tools like RevMan to quantify heterogeneity. Design cross-validation experiments to isolate confounding factors (e.g., nutrient availability in culture media) .
Q. What strategies optimize this compound’s selectivity for mitochondrial targets over off-pathway interactions?
- Methodological Answer : Use computational docking studies to predict binding affinities for mitochondrial vs. non-mitochondrial proteins. Validate selectivity via CRISPR-Cas9 knockout models of suspected off-targets. Employ proteomic profiling (e.g., affinity purification-mass spectrometry) to identify interacting partners .
Q. How to integrate multi-omics data to elucidate this compound’s systemic metabolic effects?
- Methodological Answer : Combine transcriptomic (RNA-seq), metabolomic (LC-MS), and proteomic data using pathway enrichment tools (e.g., MetaboAnalyst, STRING). Apply machine learning algorithms to identify hub genes or metabolites altered by this compound. Validate findings with targeted knock-in/knockout models .
Q. What experimental controls are critical for longitudinal studies on this compound’s chronic administration?
- Methodological Answer : Include vehicle controls, untreated cohorts, and positive controls (e.g., metformin for glucose-lowering effects). Monitor body weight, food intake, and organ histopathology. Use blinded scoring for histological analyses to reduce bias .
Q. How to address ethical and methodological challenges in translating this compound findings to preclinical trials?
- Methodological Answer : Follow ARRIVE guidelines for animal research reporting. Conduct pharmacokinetic/pharmacodynamic (PK/PD) studies to establish safe dosing ranges. Engage with regulatory bodies early to align experimental endpoints with clinical trial requirements .
Q. Data Presentation and Reproducibility Guidelines
- Tables : Include raw and normalized data (e.g., mitochondrial respiration rates under this compound treatment) with error margins and statistical significance markers (*p < 0.05) .
- Figures : Use line graphs for dose-response curves and heatmaps for omics data. Annotate all axes and provide legends for clarity .
- Supplementary Materials : Archive protocols, uncropped blots, and code for data analysis in repositories like Figshare or Zenodo .
Properties
IUPAC Name |
5-N,6-N-bis(2-fluorophenyl)-[1,2,5]oxadiazolo[3,4-b]pyrazine-5,6-diamine | |
---|---|---|
Source | PubChem | |
URL | https://pubchem.ncbi.nlm.nih.gov | |
Description | Data deposited in or computed by PubChem | |
InChI |
InChI=1S/C16H10F2N6O/c17-9-5-1-3-7-11(9)19-13-14(20-12-8-4-2-6-10(12)18)22-16-15(21-13)23-25-24-16/h1-8H,(H,19,21,23)(H,20,22,24) | |
Source | PubChem | |
URL | https://pubchem.ncbi.nlm.nih.gov | |
Description | Data deposited in or computed by PubChem | |
InChI Key |
OEGJBRZAJRPPHL-UHFFFAOYSA-N | |
Source | PubChem | |
URL | https://pubchem.ncbi.nlm.nih.gov | |
Description | Data deposited in or computed by PubChem | |
Canonical SMILES |
C1=CC=C(C(=C1)NC2=NC3=NON=C3N=C2NC4=CC=CC=C4F)F | |
Source | PubChem | |
URL | https://pubchem.ncbi.nlm.nih.gov | |
Description | Data deposited in or computed by PubChem | |
Molecular Formula |
C16H10F2N6O | |
Source | PubChem | |
URL | https://pubchem.ncbi.nlm.nih.gov | |
Description | Data deposited in or computed by PubChem | |
Molecular Weight |
340.29 g/mol | |
Source | PubChem | |
URL | https://pubchem.ncbi.nlm.nih.gov | |
Description | Data deposited in or computed by PubChem | |
Retrosynthesis Analysis
AI-Powered Synthesis Planning: Our tool employs the Template_relevance Pistachio, Template_relevance Bkms_metabolic, Template_relevance Pistachio_ringbreaker, Template_relevance Reaxys, Template_relevance Reaxys_biocatalysis model, leveraging a vast database of chemical reactions to predict feasible synthetic routes.
One-Step Synthesis Focus: Specifically designed for one-step synthesis, it provides concise and direct routes for your target compounds, streamlining the synthesis process.
Accurate Predictions: Utilizing the extensive PISTACHIO, BKMS_METABOLIC, PISTACHIO_RINGBREAKER, REAXYS, REAXYS_BIOCATALYSIS database, our tool offers high-accuracy predictions, reflecting the latest in chemical research and data.
Strategy Settings
Precursor scoring | Relevance Heuristic |
---|---|
Min. plausibility | 0.01 |
Model | Template_relevance |
Template Set | Pistachio/Bkms_metabolic/Pistachio_ringbreaker/Reaxys/Reaxys_biocatalysis |
Top-N result to add to graph | 6 |
Feasible Synthetic Routes
Disclaimer and Information on In-Vitro Research Products
Please be aware that all articles and product information presented on BenchChem are intended solely for informational purposes. The products available for purchase on BenchChem are specifically designed for in-vitro studies, which are conducted outside of living organisms. In-vitro studies, derived from the Latin term "in glass," involve experiments performed in controlled laboratory settings using cells or tissues. It is important to note that these products are not categorized as medicines or drugs, and they have not received approval from the FDA for the prevention, treatment, or cure of any medical condition, ailment, or disease. We must emphasize that any form of bodily introduction of these products into humans or animals is strictly prohibited by law. It is essential to adhere to these guidelines to ensure compliance with legal and ethical standards in research and experimentation.