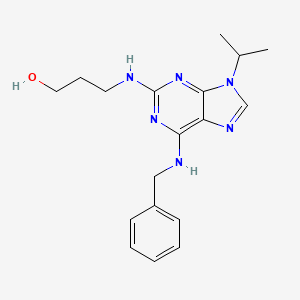
Bohemine
Overview
Description
Bohemine is a synthetic purine analogue known for its role as a cyclin-dependent kinase inhibitor. It is structurally similar to other purine analogues such as olomoucine and roscovitine. This compound has a molecular formula of C18H24N6O and a molecular weight of 340.4 g/mol . It is primarily used in scientific research due to its ability to inhibit cyclin-dependent kinases, which are crucial regulators of the cell cycle.
Mechanism of Action
Target of Action
Bohemine is a synthetic purine analogue that primarily targets cyclin-dependent kinases (Cdks), specifically Cdk2/cyclin E, Cdk2/cyclin A, and Cdk9/cyclin T1 . Cdks, in conjunction with their endogenous activator proteins known as cyclins, form the autonomous oscillator that controls the cell cycle in embryonic, somatic, and germline cells .
Mode of Action
This compound interacts with its targets (Cdks) by inhibiting their activity. The IC50 values for in vitro recombinant cdk1/Cyclin B and cdk2/Cyclin E kinase assays are 1.1 µM and 0.8 µM, respectively . This inhibition of Cdks leads to changes in the cell cycle, affecting both cell growth and division .
Biochemical Pathways
This compound’s action on Cdks affects the cell cycle, a complex biochemical pathway that regulates cell growth and division. The activity of Cdks is controlled through dephosphorylation, subcellular localization, and complex-formation with cyclins, whose expression level is tightly regulated in correspondence to cell cycle phases . Dysregulation of Cdks is known to occur in primary tumors and tumor cell lines .
Result of Action
This compound’s inhibition of Cdks results in a suppression of cell growth and an induction of cell cycle arrest at both the G1/S and G2/M boundaries . This can lead to a short-term arrest of growth and monoclonal antibody production . Interestingly, while this compound and other Cdk inhibitors typically arrest the cell cycle at the G1/S phase, in combination with ionomycin, this compound activates mature bovine oocytes to DNA synthesis and cell division .
Action Environment
The action, efficacy, and stability of this compound can be influenced by various environmental factors. For instance, the storage conditions of this compound can impact its stability, with recommendations to store it at -20°C, protected from light and air . Furthermore, the cellular environment, including the presence of other molecules like ionomycin, can influence this compound’s action as seen in the activation of mature bovine oocytes .
Biochemical Analysis
Biochemical Properties
Bohemine interacts with cyclin-dependent kinases (cdk-1 – cdk-8) and their endogenous activator proteins, cyclins . These interactions form the autonomous oscillator that controls the cell cycle in embryonic as well as somatic and germline cells in the mature animal .
Cellular Effects
This compound has been shown to have significant effects on various types of cells and cellular processes. For instance, it has been used to characterize the quantitative changes in the protein composition of the CEM T-lymphoblastic leukemia cell line .
Molecular Mechanism
This compound exerts its effects at the molecular level through its interactions with cyclin-dependent kinases and cyclins . These interactions control the activity of Cdks through dephosphorylation, subcellular localization, and complex-formation with cyclins whose expression level is tightly regulated in correspondence to cell cycle phases .
Temporal Effects in Laboratory Settings
It is known that this compound is stable and can have long-term effects on cellular function .
Metabolic Pathways
This compound is involved in the metabolic pathways that control the cell cycle. It interacts with cyclin-dependent kinases and cyclins, which play a crucial role in these pathways .
Subcellular Localization
The subcellular localization of this compound is likely to be closely linked to its interactions with cyclin-dependent kinases and cyclins
Preparation Methods
Bohemine is synthesized through a series of chemical reactions involving purine derivatives. The synthetic route typically involves the substitution of purine at specific positions with various functional groups. The preparation method includes the following steps:
Starting Material: The synthesis begins with a purine derivative.
Substitution Reactions: The purine derivative undergoes substitution reactions to introduce the (3-hydroxypropyl)amino, benzylamino, and isopropyl groups at the C-2, C-6, and N-9 positions, respectively.
Reaction Conditions: The reactions are carried out under controlled conditions, often involving the use of solvents like dimethyl sulfoxide (DMSO) and catalysts to facilitate the reactions.
Chemical Reactions Analysis
Bohemine undergoes various types of chemical reactions, including:
Oxidation: this compound can be oxidized under specific conditions to form oxidized derivatives.
Reduction: It can also undergo reduction reactions to yield reduced forms.
Substitution: this compound is involved in substitution reactions where functional groups are replaced with other groups.
Common Reagents and Conditions: Common reagents used in these reactions include oxidizing agents, reducing agents, and various catalysts. .
Scientific Research Applications
Bohemine has a wide range of applications in scientific research:
Chemistry: It is used as a model compound to study the inhibition of cyclin-dependent kinases.
Biology: this compound is employed in cell cycle studies to understand the regulation of cell division and growth.
Comparison with Similar Compounds
Bohemine is structurally and functionally similar to other purine analogues such as olomoucine and roscovitine. it has unique properties that distinguish it from these compounds:
Olomoucine: Like this compound, olomoucine is a CDK inhibitor, but it has different substitution patterns on the purine ring.
Roscovitine: Roscovitine also inhibits CDKs but has a different set of molecular targets and a distinct mechanism of action.
Similar Compounds
- Olomoucine
- Roscovitine
- Flavopiridol
- Staurosporine
- Butyrolactone
Properties
IUPAC Name |
3-[[6-(benzylamino)-9-propan-2-ylpurin-2-yl]amino]propan-1-ol | |
---|---|---|
Source | PubChem | |
URL | https://pubchem.ncbi.nlm.nih.gov | |
Description | Data deposited in or computed by PubChem | |
InChI |
InChI=1S/C18H24N6O/c1-13(2)24-12-21-15-16(20-11-14-7-4-3-5-8-14)22-18(23-17(15)24)19-9-6-10-25/h3-5,7-8,12-13,25H,6,9-11H2,1-2H3,(H2,19,20,22,23) | |
Source | PubChem | |
URL | https://pubchem.ncbi.nlm.nih.gov | |
Description | Data deposited in or computed by PubChem | |
InChI Key |
OPQGFIAVPSXOBO-UHFFFAOYSA-N | |
Source | PubChem | |
URL | https://pubchem.ncbi.nlm.nih.gov | |
Description | Data deposited in or computed by PubChem | |
Canonical SMILES |
CC(C)N1C=NC2=C(N=C(N=C21)NCCCO)NCC3=CC=CC=C3 | |
Source | PubChem | |
URL | https://pubchem.ncbi.nlm.nih.gov | |
Description | Data deposited in or computed by PubChem | |
Molecular Formula |
C18H24N6O | |
Source | PubChem | |
URL | https://pubchem.ncbi.nlm.nih.gov | |
Description | Data deposited in or computed by PubChem | |
DSSTOX Substance ID |
DTXSID00274365 | |
Record name | bohemine | |
Source | EPA DSSTox | |
URL | https://comptox.epa.gov/dashboard/DTXSID00274365 | |
Description | DSSTox provides a high quality public chemistry resource for supporting improved predictive toxicology. | |
Molecular Weight |
340.4 g/mol | |
Source | PubChem | |
URL | https://pubchem.ncbi.nlm.nih.gov | |
Description | Data deposited in or computed by PubChem | |
CAS No. |
189232-42-6 | |
Record name | Bohemine | |
Source | ChemIDplus | |
URL | https://pubchem.ncbi.nlm.nih.gov/substance/?source=chemidplus&sourceid=0189232426 | |
Description | ChemIDplus is a free, web search system that provides access to the structure and nomenclature authority files used for the identification of chemical substances cited in National Library of Medicine (NLM) databases, including the TOXNET system. | |
Record name | bohemine | |
Source | EPA DSSTox | |
URL | https://comptox.epa.gov/dashboard/DTXSID00274365 | |
Description | DSSTox provides a high quality public chemistry resource for supporting improved predictive toxicology. | |
Synthesis routes and methods
Procedure details
Retrosynthesis Analysis
AI-Powered Synthesis Planning: Our tool employs the Template_relevance Pistachio, Template_relevance Bkms_metabolic, Template_relevance Pistachio_ringbreaker, Template_relevance Reaxys, Template_relevance Reaxys_biocatalysis model, leveraging a vast database of chemical reactions to predict feasible synthetic routes.
One-Step Synthesis Focus: Specifically designed for one-step synthesis, it provides concise and direct routes for your target compounds, streamlining the synthesis process.
Accurate Predictions: Utilizing the extensive PISTACHIO, BKMS_METABOLIC, PISTACHIO_RINGBREAKER, REAXYS, REAXYS_BIOCATALYSIS database, our tool offers high-accuracy predictions, reflecting the latest in chemical research and data.
Strategy Settings
Precursor scoring | Relevance Heuristic |
---|---|
Min. plausibility | 0.01 |
Model | Template_relevance |
Template Set | Pistachio/Bkms_metabolic/Pistachio_ringbreaker/Reaxys/Reaxys_biocatalysis |
Top-N result to add to graph | 6 |
Feasible Synthetic Routes
Q1: What is the primary mechanism of action of bohemine?
A1: this compound acts as a potent inhibitor of cyclin-dependent kinases (CDKs) [, , , ]. CDKs are key regulators of the cell cycle, and their inhibition can lead to cell cycle arrest and potentially induce apoptosis, making them attractive targets for cancer therapy.
Q2: How does this compound's inhibition of CDKs affect tumor cell growth?
A2: this compound's inhibition of CDKs, particularly CDK1 and CDK2, disrupts the cell cycle progression, leading to antitumor activity both in vitro and in vivo [, ]. The inhibition of RB phosphorylation and depletion of cyclins, including cyclin D1, are observed consequences of this CDK inhibition [].
Q3: Beyond CDK inhibition, what other cellular effects have been observed with this compound?
A3: Research suggests that this compound's effects extend beyond direct CDK inhibition. Studies using proteomics approaches have identified alterations in proteins involved in metabolic pathways (glycolysis, nucleic acid synthesis, NADPH production), stress response, protein folding, cytoskeleton organization, and exocytosis [, ]. This suggests that this compound may influence various cellular processes, contributing to its overall anti-cancer effects.
Q4: What is the molecular formula and weight of this compound?
A4: The molecular formula of this compound is C20H26N6O, and its molecular weight is 366.46 g/mol.
Q5: Is there any available spectroscopic data characterizing this compound?
A5: While the provided abstracts do not detail specific spectroscopic data, studies utilize techniques like mass spectrometry and nuclear magnetic resonance (NMR) for structural elucidation of this compound and its metabolites [, ]. These techniques confirm the identity and purity of the synthesized compound.
Q6: What is known about the absorption, distribution, metabolism, and excretion (ADME) of this compound?
A8: In mice, this compound is rapidly cleared from circulation after intravenous administration, with metabolites identified in both bile and urine []. The primary metabolic route is glucosidation at the terminal hydroxyl group, suggesting significant hepatic metabolism. Further research is needed to fully elucidate its ADME profile in other species, including humans.
Q7: How do the in vivo pharmacokinetic properties of this compound compare to its in vitro activity?
A9: While this compound exhibits potent in vitro activity against various cancer cell lines, its rapid in vivo metabolism suggests a potential discrepancy between in vitro and in vivo efficacy [, ]. This highlights the importance of considering both pharmacokinetic and pharmacodynamic properties in drug development.
Q8: What types of in vitro assays have been used to assess this compound's activity?
A10: Researchers have employed various in vitro assays, including cell proliferation assays (sulforhodamine B assay) and kinase activity assays, to determine this compound's potency and selectivity against CDKs and its impact on cancer cell growth [, , ].
Q9: Has this compound demonstrated efficacy in any in vivo models?
A11: While the provided abstracts do not directly demonstrate this compound's in vivo efficacy, studies utilizing its structural analog, CYC202, in mouse xenograft models show tumor growth inhibition and modulation of pharmacodynamic markers []. This suggests that this compound, with further optimization, might also demonstrate in vivo efficacy.
Q10: Have any resistance mechanisms to this compound been identified?
A12: While specific resistance mechanisms to this compound are not explicitly discussed in the provided abstracts, a study using a this compound-resistant CEM T-lymphoblastic leukemia cell line identified potential protein targets associated with resistance development []. These include Rho GDP-dissociation inhibitor 2, Y-box binding protein 1, and the HSP70/90 organizing protein, suggesting their potential involvement in conferring resistance to CDK inhibitors like this compound.
Q11: What is the current understanding of this compound's toxicity profile?
A11: Information on this compound's toxicity and safety profile is limited within the provided abstracts. Further research is needed to thoroughly evaluate its potential toxicity and long-term effects.
Q12: How does this compound's structure influence its activity and selectivity?
A14: While specific SAR studies are not detailed in the abstracts, the development of this compound from olomoucine and the comparison with other analogs like CYC202 highlight the impact of structural modifications on CDK inhibitory activity, potency, and selectivity [, , ]. Modifications to the substituents at positions 2, 6, and 9 of the purine ring significantly influence these properties.
Q13: Are there alternative compounds or strategies being explored that target similar pathways or mechanisms as this compound?
A16: The development of various CDK inhibitors, including CYC202 (seliciclib), which has progressed to clinical trials [], highlights the active exploration of alternative compounds targeting CDKs as potential cancer therapies.
Disclaimer and Information on In-Vitro Research Products
Please be aware that all articles and product information presented on BenchChem are intended solely for informational purposes. The products available for purchase on BenchChem are specifically designed for in-vitro studies, which are conducted outside of living organisms. In-vitro studies, derived from the Latin term "in glass," involve experiments performed in controlled laboratory settings using cells or tissues. It is important to note that these products are not categorized as medicines or drugs, and they have not received approval from the FDA for the prevention, treatment, or cure of any medical condition, ailment, or disease. We must emphasize that any form of bodily introduction of these products into humans or animals is strictly prohibited by law. It is essential to adhere to these guidelines to ensure compliance with legal and ethical standards in research and experimentation.