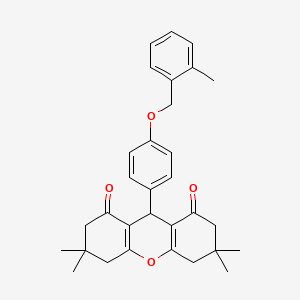
3,3,6,6-Tetramethyl-9-(4-((2-methylbenzyl)oxy)phenyl)-3,4,5,6,7,9-hexahydro-1H-xanthene-1,8(2H)-dione
Overview
Description
BMS 986187 is a positive allosteric modulator of δ-opioid receptors. In the presence of the endogenous δ-opioid receptor agonist leu-enkephalin, BMS 986187 has an average EC50 value of 30 nM for β-arrestin recruitment, but has little to no activity in the absence of an agonist. It is 100-fold selective for δ- over µ-opioid receptors in the presence of leu-enkephalin and the endogenous µ-opioid receptor agonist endomorphin I, respectively.
BMS-986187 is a novel Potent and Selective Positive Allosteric Modulators of the delta-Opioid Receptor.
Scientific Research Applications
Antimicrobial Properties
- A study synthesized novel derivatives of this compound and found them to exhibit good to excellent antibacterial and antifungal activities in an agar well diffusion assay (Angajala et al., 2017).
Anticancer Potential
- Research demonstrated that some derivatives showed promising anti-proliferative properties against cancer cell lines, indicating potential as anticancer agents (Mulakayala et al., 2012).
Structural and Spectroscopic Analysis
- Comparative analysis on derivatives of this compound provided insights into their structural geometry and potential pharmacological importance, based on molecular docking studies (Kumar et al., 2020).
Synthesis Techniques
- A simple, environmentally friendly one-pot reaction for synthesizing a related compound was reported, demonstrating an advancement in synthesis techniques (Navarro et al., 2016).
Antioxidant and Antimicrobial Activities
- Synthesized derivatives of this compound were evaluated for antioxidant, antimicrobial, and antiproliferative activities, showing potential as antioxidant and antiproliferative agents (Zukić et al., 2018).
Vanillin Derivative Compounds
- Study of vanillin derivative compounds related to this chemical structure revealed strong antibacterial activity against various bacteria (Retnosari et al., 2021).
Intermolecular Interactions
- Research on xanthenedione molecules, closely related to the compound , highlighted their intermolecular interactions and biological activity potential, especially as antioxidants and acetylcholinesterase inhibitors (Purushothaman & Thiruvenkatam, 2018).
Mechanism of Action
Target of Action
BMS-986187, also known as 3,3,6,6-Tetramethyl-9-(4-((2-methylbenzyl)oxy)phenyl)-3,4,5,6,7,9-hexahydro-1H-xanthene-1,8(2H)-dione, is a selective positive allosteric modulator (PAM) of the δ-opioid receptor . The δ-opioid receptor is an emerging target for the management of chronic pain and depression .
Mode of Action
BMS-986187 interacts with its target, the δ-opioid receptor, by enhancing the receptor’s response to its natural ligands . This interaction results in a change in the receptor’s conformation, which leads to the activation of intracellular signaling pathways . BMS-986187 exhibits 100-fold selectivity for the δ-opioid receptor over the μ-opioid receptor .
Biochemical Pathways
The δ-opioid receptor is part of the G-protein-coupled receptor (GPCR) family. When BMS-986187 binds to the δ-opioid receptor, it enhances the receptor’s response to its natural ligands, leading to the activation of G-protein signaling pathways . This biased signaling, the preferential activation of one signaling pathway over another downstream of δ-receptors, may generate better therapeutic profiles .
Pharmacokinetics
A study on a similar compound, bms-986177, showed that hepatic impairment could affect the pharmacokinetic properties of the compound
Result of Action
BMS-986187 enhances the affinity of natural ligands for the δ-opioid receptor . This results in the activation of intracellular signaling pathways, which can lead to various cellular responses.
Action Environment
The action of BMS-986187 can be influenced by various environmental factors. For instance, the presence of natural ligands can enhance the compound’s activity . Additionally, a study suggested that BMS-986187 is sensitive to allosteric modulation with different chemical scaffolds
properties
IUPAC Name |
3,3,6,6-tetramethyl-9-[4-[(2-methylphenyl)methoxy]phenyl]-4,5,7,9-tetrahydro-2H-xanthene-1,8-dione | |
---|---|---|
Source | PubChem | |
URL | https://pubchem.ncbi.nlm.nih.gov | |
Description | Data deposited in or computed by PubChem | |
InChI |
InChI=1S/C31H34O4/c1-19-8-6-7-9-21(19)18-34-22-12-10-20(11-13-22)27-28-23(32)14-30(2,3)16-25(28)35-26-17-31(4,5)15-24(33)29(26)27/h6-13,27H,14-18H2,1-5H3 | |
Source | PubChem | |
URL | https://pubchem.ncbi.nlm.nih.gov | |
Description | Data deposited in or computed by PubChem | |
InChI Key |
UEKIYVKPQNKSDI-UHFFFAOYSA-N | |
Source | PubChem | |
URL | https://pubchem.ncbi.nlm.nih.gov | |
Description | Data deposited in or computed by PubChem | |
Canonical SMILES |
CC1=CC=CC=C1COC2=CC=C(C=C2)C3C4=C(CC(CC4=O)(C)C)OC5=C3C(=O)CC(C5)(C)C | |
Source | PubChem | |
URL | https://pubchem.ncbi.nlm.nih.gov | |
Description | Data deposited in or computed by PubChem | |
Molecular Formula |
C31H34O4 | |
Source | PubChem | |
URL | https://pubchem.ncbi.nlm.nih.gov | |
Description | Data deposited in or computed by PubChem | |
Molecular Weight |
470.6 g/mol | |
Source | PubChem | |
URL | https://pubchem.ncbi.nlm.nih.gov | |
Description | Data deposited in or computed by PubChem | |
Retrosynthesis Analysis
AI-Powered Synthesis Planning: Our tool employs the Template_relevance Pistachio, Template_relevance Bkms_metabolic, Template_relevance Pistachio_ringbreaker, Template_relevance Reaxys, Template_relevance Reaxys_biocatalysis model, leveraging a vast database of chemical reactions to predict feasible synthetic routes.
One-Step Synthesis Focus: Specifically designed for one-step synthesis, it provides concise and direct routes for your target compounds, streamlining the synthesis process.
Accurate Predictions: Utilizing the extensive PISTACHIO, BKMS_METABOLIC, PISTACHIO_RINGBREAKER, REAXYS, REAXYS_BIOCATALYSIS database, our tool offers high-accuracy predictions, reflecting the latest in chemical research and data.
Strategy Settings
Precursor scoring | Relevance Heuristic |
---|---|
Min. plausibility | 0.01 |
Model | Template_relevance |
Template Set | Pistachio/Bkms_metabolic/Pistachio_ringbreaker/Reaxys/Reaxys_biocatalysis |
Top-N result to add to graph | 6 |
Feasible Synthetic Routes
Q & A
Q1: What makes BMS-986187 a unique therapeutic approach for potential treatment of conditions like IBS?
A1: Unlike traditional agonists that bind directly to the active site of the δ-opioid receptor (DOR), BMS-986187 acts as a positive allosteric modulator (PAM) [, , , , , ]. This means it binds to a different site on the DOR, enhancing the receptor's response to naturally occurring agonists like enkephalins. This approach offers potential advantages like increased selectivity, potentially leading to fewer side effects []. Research suggests that BMS-986187 might be beneficial in treating gut motility disorders like IBS by augmenting the inhibitory effects of DOR agonists on neurogenic contractions in the enteric nervous system (ENS) [].
Q2: How does BMS-986187 impact DOR signaling within the enteric nervous system?
A2: BMS-986187 demonstrates probe-dependence in its actions on DOR in the mouse colon, meaning its effects are contingent upon the presence of an orthosteric agonist []. It enhances the effects of DOR agonists by increasing their ability to inhibit neurogenic contractions, suggesting a potentiation of the DOR signaling pathway []. Additionally, BMS-986187 promotes DOR internalization in myenteric neurons, even in response to weakly internalizing agonists like ARM390, further indicating its influence on DOR signaling dynamics [].
Q3: Can BMS-986187 directly activate the δ-opioid receptor without an orthosteric agonist present?
A3: Research indicates that BMS-986187 can indeed function as a G-protein-biased allosteric agonist []. This means that it can directly activate the DOR, even in the absence of a primary agonist bound to the orthosteric site, and preferentially activate G protein signaling pathways []. This direct activation capability, coupled with its biased signaling, underscores the potential of BMS-986187 as a unique therapeutic tool.
Q4: What are the implications of BMS-986187's ability to enhance endogenous opioid activity for pain management?
A4: Studies have shown that BMS-986187, by itself, can produce significant antinociception, likely by amplifying the actions of naturally occurring opioids in the body []. Furthermore, it can potentiate the analgesic effects of certain opioid agonists, such as methadone, in a dose- and time-dependent manner []. These findings highlight the potential of BMS-986187 to achieve effective pain relief by leveraging and boosting the body's innate pain-modulating mechanisms.
Q5: Has BMS-986187 demonstrated any potential in treating conditions beyond pain and IBS?
A5: Preclinical studies suggest that BMS-986187 may hold promise in treating depression [, ]. It demonstrates antidepressant-like effects in mice, which are blocked by the DOR antagonist naltrindole and absent in DOR knockout mice, confirming a DOR-mediated mechanism [, ]. Furthermore, the antidepressant effects of BMS-986187 appear to be potentiated in the absence of μ-opioid receptor (MOR) activity, suggesting a complex interplay between DOR and MOR in mediating these effects [].
Disclaimer and Information on In-Vitro Research Products
Please be aware that all articles and product information presented on BenchChem are intended solely for informational purposes. The products available for purchase on BenchChem are specifically designed for in-vitro studies, which are conducted outside of living organisms. In-vitro studies, derived from the Latin term "in glass," involve experiments performed in controlled laboratory settings using cells or tissues. It is important to note that these products are not categorized as medicines or drugs, and they have not received approval from the FDA for the prevention, treatment, or cure of any medical condition, ailment, or disease. We must emphasize that any form of bodily introduction of these products into humans or animals is strictly prohibited by law. It is essential to adhere to these guidelines to ensure compliance with legal and ethical standards in research and experimentation.