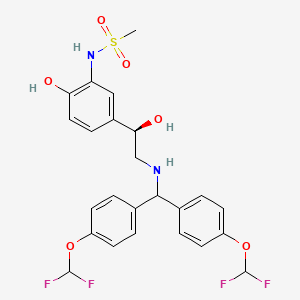
BMS-194449
Overview
Description
BMS-194449 is a chemical compound known for its role as a beta 3 receptor agonist. The compound has a molecular formula of C24H24F4N2O6S and a molecular weight of 544.52 .
Preparation Methods
The synthesis of BMS-194449 involves several steps :
Nitration: 4-Hydroxyacetophenone is nitrated with potassium nitrate in cold sulfuric acid to produce the 3-nitro derivative.
Protection: The nitro derivative is protected as the benzyl ether using benzyl bromide in dimethylformamide.
Hydrogenation: The nitro group is reduced to aniline using platinum oxide.
Sulfonamide Formation: The aniline is treated with methanesulfonyl chloride in pyridine to form the sulfonamide.
Bromination: The acetophenone is brominated using copper bromide in a refluxing mixture of ethyl acetate and chloroform.
Asymmetric Reduction: The ketone is reduced with borane in the presence of a chiral oxazaborolidine to produce the ®-alcohol.
Substitution: The bromo group is substituted with an iodo group using sodium iodide in acetone.
Protection: The resulting compound is protected as the triethylsilyl ether using triethylsilyl chloride in the presence of imidazole and dimethylaminopyridine.
Condensation: The iodide is condensed with benzhydryl amine in the presence of diisopropyl ethylamine in tetrahydrofuran.
Desilylation and Hydrogenolysis: The final compound is obtained by desilylation with tetrabutylammonium fluoride, followed by hydrogenolysis of the benzyl protecting group.
Chemical Reactions Analysis
BMS-194449 undergoes several types of chemical reactions :
Oxidation and Reduction: The nitro group is reduced to aniline, and the ketone is asymmetrically reduced to an alcohol.
Substitution: The bromo group is substituted with an iodo group.
Protection and Deprotection: The compound undergoes protection as benzyl ether and triethylsilyl ether, followed by deprotection.
Common reagents used in these reactions include potassium nitrate, sulfuric acid, benzyl bromide, platinum oxide, methanesulfonyl chloride, copper bromide, borane, sodium iodide, triethylsilyl chloride, imidazole, dimethylaminopyridine, diisopropyl ethylamine, and tetrabutylammonium fluoride .
Scientific Research Applications
Chemistry: It serves as a model compound for studying beta 3 receptor agonists.
Biology: It is used to investigate the biological pathways involving beta 3 receptors.
Medicine: BMS-194449 is explored for its potential in treating obesity and non-insulin dependent diabetes.
Industry: It is used in the development of new therapeutic agents targeting beta 3 receptors.
Mechanism of Action
BMS-194449 exerts its effects by acting as a beta 3 receptor agonist . It binds to beta 3 adrenergic receptors, which are involved in the regulation of energy expenditure and lipolysis. By activating these receptors, this compound promotes the breakdown of fats and increases energy expenditure, making it a potential therapeutic agent for obesity and diabetes .
Comparison with Similar Compounds
BMS-194449 is unique among beta 3 receptor agonists due to its specific chemical structure and high selectivity for beta 3 receptors . Similar compounds include:
Mirabegron: Another beta 3 receptor agonist used for treating overactive bladder.
Solabegron: A beta 3 receptor agonist studied for its potential in treating irritable bowel syndrome.
BRL 37344: A beta 3 receptor agonist used in research for its effects on metabolism and energy expenditure.
This compound stands out due to its specific applications in obesity and diabetes research, as well as its unique synthetic route and chemical properties .
Properties
IUPAC Name |
N-[5-[(1R)-2-[bis[4-(difluoromethoxy)phenyl]methylamino]-1-hydroxyethyl]-2-hydroxyphenyl]methanesulfonamide | |
---|---|---|
Source | PubChem | |
URL | https://pubchem.ncbi.nlm.nih.gov | |
Description | Data deposited in or computed by PubChem | |
InChI |
InChI=1S/C24H24F4N2O6S/c1-37(33,34)30-19-12-16(6-11-20(19)31)21(32)13-29-22(14-2-7-17(8-3-14)35-23(25)26)15-4-9-18(10-5-15)36-24(27)28/h2-12,21-24,29-32H,13H2,1H3/t21-/m0/s1 | |
Source | PubChem | |
URL | https://pubchem.ncbi.nlm.nih.gov | |
Description | Data deposited in or computed by PubChem | |
InChI Key |
FUKHGCVTMAEHRG-NRFANRHFSA-N | |
Source | PubChem | |
URL | https://pubchem.ncbi.nlm.nih.gov | |
Description | Data deposited in or computed by PubChem | |
Canonical SMILES |
CS(=O)(=O)NC1=C(C=CC(=C1)C(CNC(C2=CC=C(C=C2)OC(F)F)C3=CC=C(C=C3)OC(F)F)O)O | |
Source | PubChem | |
URL | https://pubchem.ncbi.nlm.nih.gov | |
Description | Data deposited in or computed by PubChem | |
Isomeric SMILES |
CS(=O)(=O)NC1=C(C=CC(=C1)[C@H](CNC(C2=CC=C(C=C2)OC(F)F)C3=CC=C(C=C3)OC(F)F)O)O | |
Source | PubChem | |
URL | https://pubchem.ncbi.nlm.nih.gov | |
Description | Data deposited in or computed by PubChem | |
Molecular Formula |
C24H24F4N2O6S | |
Source | PubChem | |
URL | https://pubchem.ncbi.nlm.nih.gov | |
Description | Data deposited in or computed by PubChem | |
DSSTOX Substance ID |
DTXSID301108692 | |
Record name | N-[5-[(1R)-2-[[Bis[4-(difluoromethoxy)phenyl]methyl]amino]-1-hydroxyethyl]-2-hydroxyphenyl]methanesulfonamide | |
Source | EPA DSSTox | |
URL | https://comptox.epa.gov/dashboard/DTXSID301108692 | |
Description | DSSTox provides a high quality public chemistry resource for supporting improved predictive toxicology. | |
Molecular Weight |
544.5 g/mol | |
Source | PubChem | |
URL | https://pubchem.ncbi.nlm.nih.gov | |
Description | Data deposited in or computed by PubChem | |
CAS No. |
170686-12-1 | |
Record name | N-[5-[(1R)-2-[[Bis[4-(difluoromethoxy)phenyl]methyl]amino]-1-hydroxyethyl]-2-hydroxyphenyl]methanesulfonamide | |
Source | CAS Common Chemistry | |
URL | https://commonchemistry.cas.org/detail?cas_rn=170686-12-1 | |
Description | CAS Common Chemistry is an open community resource for accessing chemical information. Nearly 500,000 chemical substances from CAS REGISTRY cover areas of community interest, including common and frequently regulated chemicals, and those relevant to high school and undergraduate chemistry classes. This chemical information, curated by our expert scientists, is provided in alignment with our mission as a division of the American Chemical Society. | |
Explanation | The data from CAS Common Chemistry is provided under a CC-BY-NC 4.0 license, unless otherwise stated. | |
Record name | BMS-194449 | |
Source | ChemIDplus | |
URL | https://pubchem.ncbi.nlm.nih.gov/substance/?source=chemidplus&sourceid=0170686121 | |
Description | ChemIDplus is a free, web search system that provides access to the structure and nomenclature authority files used for the identification of chemical substances cited in National Library of Medicine (NLM) databases, including the TOXNET system. | |
Record name | N-[5-[(1R)-2-[[Bis[4-(difluoromethoxy)phenyl]methyl]amino]-1-hydroxyethyl]-2-hydroxyphenyl]methanesulfonamide | |
Source | EPA DSSTox | |
URL | https://comptox.epa.gov/dashboard/DTXSID301108692 | |
Description | DSSTox provides a high quality public chemistry resource for supporting improved predictive toxicology. | |
Record name | BMS-194449 | |
Source | FDA Global Substance Registration System (GSRS) | |
URL | https://gsrs.ncats.nih.gov/ginas/app/beta/substances/93ZDV2P17I | |
Description | The FDA Global Substance Registration System (GSRS) enables the efficient and accurate exchange of information on what substances are in regulated products. Instead of relying on names, which vary across regulatory domains, countries, and regions, the GSRS knowledge base makes it possible for substances to be defined by standardized, scientific descriptions. | |
Explanation | Unless otherwise noted, the contents of the FDA website (www.fda.gov), both text and graphics, are not copyrighted. They are in the public domain and may be republished, reprinted and otherwise used freely by anyone without the need to obtain permission from FDA. Credit to the U.S. Food and Drug Administration as the source is appreciated but not required. | |
Retrosynthesis Analysis
AI-Powered Synthesis Planning: Our tool employs the Template_relevance Pistachio, Template_relevance Bkms_metabolic, Template_relevance Pistachio_ringbreaker, Template_relevance Reaxys, Template_relevance Reaxys_biocatalysis model, leveraging a vast database of chemical reactions to predict feasible synthetic routes.
One-Step Synthesis Focus: Specifically designed for one-step synthesis, it provides concise and direct routes for your target compounds, streamlining the synthesis process.
Accurate Predictions: Utilizing the extensive PISTACHIO, BKMS_METABOLIC, PISTACHIO_RINGBREAKER, REAXYS, REAXYS_BIOCATALYSIS database, our tool offers high-accuracy predictions, reflecting the latest in chemical research and data.
Strategy Settings
Precursor scoring | Relevance Heuristic |
---|---|
Min. plausibility | 0.01 |
Model | Template_relevance |
Template Set | Pistachio/Bkms_metabolic/Pistachio_ringbreaker/Reaxys/Reaxys_biocatalysis |
Top-N result to add to graph | 6 |
Feasible Synthetic Routes
Disclaimer and Information on In-Vitro Research Products
Please be aware that all articles and product information presented on BenchChem are intended solely for informational purposes. The products available for purchase on BenchChem are specifically designed for in-vitro studies, which are conducted outside of living organisms. In-vitro studies, derived from the Latin term "in glass," involve experiments performed in controlled laboratory settings using cells or tissues. It is important to note that these products are not categorized as medicines or drugs, and they have not received approval from the FDA for the prevention, treatment, or cure of any medical condition, ailment, or disease. We must emphasize that any form of bodily introduction of these products into humans or animals is strictly prohibited by law. It is essential to adhere to these guidelines to ensure compliance with legal and ethical standards in research and experimentation.