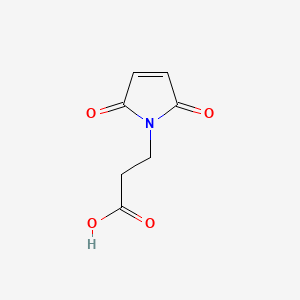
3-Maleimidopropionic acid
Overview
Description
3-Maleimidopropionic acid is an organic compound with the molecular formula C7H7NO4. It contains a maleimide group and a terminal carboxylic acid. This compound is known for its reactivity with thiol groups, making it a valuable reagent in various chemical and biological applications .
Mechanism of Action
Target of Action
3-Maleimidopropionic acid (3MP) is primarily used in the synthesis of Proteolysis Targeting Chimeras (PROTACs) . PROTACs are a class of drugs that work by recruiting an E3 ubiquitin ligase to a target protein, leading to the degradation of the target protein .
Mode of Action
3MP contains a maleimide group and a terminal carboxylic acid . The terminal carboxylic acid can react with primary amine groups in the presence of activators (e.g., EDC, or HATU) to form a stable amide bond . The maleimide group will react with a thiol group to form a covalent bond, enabling the connection of biomolecules .
Biochemical Pathways
3MP, as a component of PROTACs, exploits the intracellular ubiquitin-proteasome system to selectively degrade target proteins . This process involves the tagging of the target protein with ubiquitin molecules, which signals the proteasome to degrade the tagged protein .
Pharmacokinetics
It is known to be a component of the stabilizing solution for ec145, a folate-targeted vinca alkaloid conjugate, used in rodent pharmacokinetic studies .
Result of Action
The primary result of 3MP’s action is the degradation of target proteins when it is used in the synthesis of PROTACs . Additionally, 3MP has been shown to inhibit the production of reactive oxygen species, which are produced by cancer cells as a result of chemotherapy . It also has potent inhibitory activity against bacterial organisms such as methicillin-resistant Staphylococcus aureus (MRSA) and Clostridium perfringens, and antiviral properties against HIV infection .
Action Environment
The action of 3MP is influenced by environmental factors such as temperature and pH. For instance, it is recommended to be stored at 2-8°C . Moreover, the solubility of 3MP can be affected by the solvent used, which can impact its bioavailability and efficacy .
Preparation Methods
3-Maleimidopropionic acid can be synthesized through the reaction of maleic anhydride with β-alanine in acetic acid. The mixture is stirred at room temperature, followed by heating to 115°C overnight . This method yields a white to pale yellow solid, which is the desired product.
Chemical Reactions Analysis
3-Maleimidopropionic acid undergoes several types of chemical reactions:
Substitution Reactions: The maleimide group reacts with thiol groups to form covalent bonds, enabling the connection of biomolecules with thiol groups.
Amide Bond Formation: The terminal carboxylic acid can react with primary amine groups in the presence of activators like EDC or HATU to form stable amide bonds.
Oxidation and Reduction: While specific oxidation and reduction reactions are not commonly reported, the compound’s functional groups suggest potential reactivity under appropriate conditions.
Scientific Research Applications
3-Maleimidopropionic acid has a wide range of applications in scientific research:
Comparison with Similar Compounds
3-Maleimidopropionic acid is unique due to its dual functional groups, which enable it to react with both thiol and amine groups. Similar compounds include:
N-(3-Maleimidopropionyloxy)succinimide: This compound also contains a maleimide group and is used as a crosslinking reagent.
N-Maleoyl-β-alanine N-hydroxysuccinimide ester: Another similar compound with applications in protein modification.
Properties
IUPAC Name |
3-(2,5-dioxopyrrol-1-yl)propanoic acid | |
---|---|---|
Source | PubChem | |
URL | https://pubchem.ncbi.nlm.nih.gov | |
Description | Data deposited in or computed by PubChem | |
InChI |
InChI=1S/C7H7NO4/c9-5-1-2-6(10)8(5)4-3-7(11)12/h1-2H,3-4H2,(H,11,12) | |
Source | PubChem | |
URL | https://pubchem.ncbi.nlm.nih.gov | |
Description | Data deposited in or computed by PubChem | |
InChI Key |
IUTPJBLLJJNPAJ-UHFFFAOYSA-N | |
Source | PubChem | |
URL | https://pubchem.ncbi.nlm.nih.gov | |
Description | Data deposited in or computed by PubChem | |
Canonical SMILES |
C1=CC(=O)N(C1=O)CCC(=O)O | |
Source | PubChem | |
URL | https://pubchem.ncbi.nlm.nih.gov | |
Description | Data deposited in or computed by PubChem | |
Molecular Formula |
C7H7NO4 | |
Source | PubChem | |
URL | https://pubchem.ncbi.nlm.nih.gov | |
Description | Data deposited in or computed by PubChem | |
DSSTOX Substance ID |
DTXSID00341526 | |
Record name | 3-Maleimidopropionic acid | |
Source | EPA DSSTox | |
URL | https://comptox.epa.gov/dashboard/DTXSID00341526 | |
Description | DSSTox provides a high quality public chemistry resource for supporting improved predictive toxicology. | |
Molecular Weight |
169.13 g/mol | |
Source | PubChem | |
URL | https://pubchem.ncbi.nlm.nih.gov | |
Description | Data deposited in or computed by PubChem | |
CAS No. |
7423-55-4 | |
Record name | 3-Maleimidopropionic acid | |
Source | CAS Common Chemistry | |
URL | https://commonchemistry.cas.org/detail?cas_rn=7423-55-4 | |
Description | CAS Common Chemistry is an open community resource for accessing chemical information. Nearly 500,000 chemical substances from CAS REGISTRY cover areas of community interest, including common and frequently regulated chemicals, and those relevant to high school and undergraduate chemistry classes. This chemical information, curated by our expert scientists, is provided in alignment with our mission as a division of the American Chemical Society. | |
Explanation | The data from CAS Common Chemistry is provided under a CC-BY-NC 4.0 license, unless otherwise stated. | |
Record name | 3-Maleimidopropionic acid | |
Source | EPA DSSTox | |
URL | https://comptox.epa.gov/dashboard/DTXSID00341526 | |
Description | DSSTox provides a high quality public chemistry resource for supporting improved predictive toxicology. | |
Synthesis routes and methods I
Procedure details
Synthesis routes and methods II
Procedure details
Retrosynthesis Analysis
AI-Powered Synthesis Planning: Our tool employs the Template_relevance Pistachio, Template_relevance Bkms_metabolic, Template_relevance Pistachio_ringbreaker, Template_relevance Reaxys, Template_relevance Reaxys_biocatalysis model, leveraging a vast database of chemical reactions to predict feasible synthetic routes.
One-Step Synthesis Focus: Specifically designed for one-step synthesis, it provides concise and direct routes for your target compounds, streamlining the synthesis process.
Accurate Predictions: Utilizing the extensive PISTACHIO, BKMS_METABOLIC, PISTACHIO_RINGBREAKER, REAXYS, REAXYS_BIOCATALYSIS database, our tool offers high-accuracy predictions, reflecting the latest in chemical research and data.
Strategy Settings
Precursor scoring | Relevance Heuristic |
---|---|
Min. plausibility | 0.01 |
Model | Template_relevance |
Template Set | Pistachio/Bkms_metabolic/Pistachio_ringbreaker/Reaxys/Reaxys_biocatalysis |
Top-N result to add to graph | 6 |
Feasible Synthetic Routes
Disclaimer and Information on In-Vitro Research Products
Please be aware that all articles and product information presented on BenchChem are intended solely for informational purposes. The products available for purchase on BenchChem are specifically designed for in-vitro studies, which are conducted outside of living organisms. In-vitro studies, derived from the Latin term "in glass," involve experiments performed in controlled laboratory settings using cells or tissues. It is important to note that these products are not categorized as medicines or drugs, and they have not received approval from the FDA for the prevention, treatment, or cure of any medical condition, ailment, or disease. We must emphasize that any form of bodily introduction of these products into humans or animals is strictly prohibited by law. It is essential to adhere to these guidelines to ensure compliance with legal and ethical standards in research and experimentation.