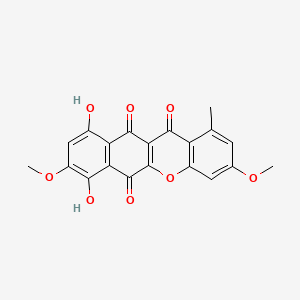
Bikaverin
Overview
Description
Bikaverin is a reddish pigment produced by various fungal species, predominantly from the genus Fusarium. It is a polyketide with a tetracyclic benzoxanthone structure, known for its antibiotic properties against certain protozoa and fungi . The compound has garnered significant interest due to its diverse biological activities, including antitumoral properties .
Mechanism of Action
Target of Action
Bikaverin, also known as Lycopersin, is a reddish pigment produced by different fungal species, most notably those from the genus Fusarium . It exhibits antibiotic properties against certain protozoa and fungi . The primary targets of this compound are cancer cell lines, against which it has demonstrated antitumoral activity .
Mode of Action
It is known that naphthoquinones, the class of compounds to which this compound belongs, interact with mitochondria and cytosolic proteins, acting as free radical carriers . The proposed mechanism is that flavoproteins catalyze electron flow from NAD(P)H to O2, producing a free radical pigment .
Pharmacokinetics
It is known that the production of this compound by different fungal species depends on culture conditions, and it is mainly affected by nitrogen availability and ph .
Result of Action
This compound has shown antitumor effects against different cancer cell lines . It also presents antimicrobial and antifungal activities . In addition to its colorant properties, this compound has shown biological activity against the protozoan Leishmania brasiliensis, the oomycete Phytophtora infestans, and the nematode Bursaphelenchus xylophilus .
Action Environment
The action of this compound is influenced by environmental factors. Its production is mainly affected by nitrogen availability and pH . The synthesis is induced by nitrogen starvation and acidic pH, and it is favored by other factors, such as aeration, sulfate and phosphate starvation, or sucrose availability . These factors increase mRNA levels of the enzymatic genes, organized in a coregulated cluster .
Safety and Hazards
Future Directions
Biochemical Analysis
Biochemical Properties
Chemically, Bikaverin is a polyketide with a tetracyclic benzoxanthone structure . This structure results from the activity of a specific class I multifunctional polyketide synthase and subsequent group modifications introduced by a monooxygenase and an O-methyltransferase .
Cellular Effects
The biological properties of this compound include antitumoral activity against different cancer cell lines . This suggests that this compound may influence cell function, including impacts on cell signaling pathways, gene expression, and cellular metabolism.
Molecular Mechanism
This compound exerts its effects at the molecular level through its interactions with various biomolecules. It is biosynthesized by the condensation of nine acetate units in a single polyketide chain, which is then folded and cross-linked to form the backbone of the molecule .
Temporal Effects in Laboratory Settings
Its production by different fungal species depends on culture conditions, but it is mainly affected by nitrogen availability and pH .
Metabolic Pathways
This compound is involved in specific metabolic pathways related to the synthesis of polyketides . These pathways involve the action of multifunctional polyketide synthase enzymes .
Preparation Methods
Synthetic Routes and Reaction Conditions: Bikaverin is primarily produced through fermentation processes involving fungal species such as Fusarium oxysporum and Fusarium fujikuroi . The production is influenced by several factors, including nitrogen availability, pH, aeration, and the presence of specific nutrients like sulfate and phosphate . Optimal conditions for this compound production include nitrogen starvation and acidic pH .
Industrial Production Methods: Industrial production of this compound involves optimizing fermentation conditions to maximize yield. Parameters such as agitation speed, temperature, and substrate concentration are crucial . For instance, maximum production has been observed at an agitation speed of 289 rpm, a temperature of 24.3°C, and a rice concentration of 16.4 g/L in the culture medium .
Chemical Reactions Analysis
Types of Reactions: Bikaverin undergoes various chemical reactions, including oxidation, reduction, and substitution . These reactions are essential for modifying the compound to enhance its biological activities or to produce derivatives with specific properties.
Common Reagents and Conditions: Common reagents used in this compound reactions include oxidizing agents like hydrogen peroxide and reducing agents such as sodium borohydride . The reactions typically occur under controlled conditions to ensure the desired modifications are achieved without degrading the compound.
Major Products: The major products formed from this compound reactions depend on the specific reagents and conditions used. For example, oxidation can lead to the formation of quinone derivatives, while reduction might produce hydroquinone derivatives .
Scientific Research Applications
Bikaverin has a wide range of applications in scientific research due to its unique properties :
Chemistry: Used as a model compound for studying polyketide biosynthesis and for developing synthetic analogs with enhanced properties.
Biology: Investigated for its role in fungal metabolism and its interactions with other microbial species.
Medicine: Explored for its antitumoral, antimicrobial, and antiparasitic activities.
Comparison with Similar Compounds
Bikaverin is unique among polyketides due to its specific structure and biological activities . Similar compounds include:
Fusarubin: Another red pigment produced by Fusarium species, known for its antibiotic properties.
Northis compound: A precursor molecule to this compound, found in smaller amounts in some fungi.
Passiflorin and Mycogonin: Pigments with similar structures but different biological activities.
Properties
IUPAC Name |
7,10-dihydroxy-3,8-dimethoxy-1-methylbenzo[b]xanthene-6,11,12-trione | |
---|---|---|
Source | PubChem | |
URL | https://pubchem.ncbi.nlm.nih.gov | |
Description | Data deposited in or computed by PubChem | |
InChI |
InChI=1S/C20H14O8/c1-7-4-8(26-2)5-10-12(7)17(23)15-18(24)13-9(21)6-11(27-3)16(22)14(13)19(25)20(15)28-10/h4-6,21-22H,1-3H3 | |
Source | PubChem | |
URL | https://pubchem.ncbi.nlm.nih.gov | |
Description | Data deposited in or computed by PubChem | |
InChI Key |
QXNACSREWQXWCV-UHFFFAOYSA-N | |
Source | PubChem | |
URL | https://pubchem.ncbi.nlm.nih.gov | |
Description | Data deposited in or computed by PubChem | |
Canonical SMILES |
CC1=CC(=CC2=C1C(=O)C3=C(O2)C(=O)C4=C(C3=O)C(=CC(=C4O)OC)O)OC | |
Source | PubChem | |
URL | https://pubchem.ncbi.nlm.nih.gov | |
Description | Data deposited in or computed by PubChem | |
Molecular Formula |
C20H14O8 | |
Source | PubChem | |
URL | https://pubchem.ncbi.nlm.nih.gov | |
Description | Data deposited in or computed by PubChem | |
DSSTOX Substance ID |
DTXSID80187020 | |
Record name | Bikaverin | |
Source | EPA DSSTox | |
URL | https://comptox.epa.gov/dashboard/DTXSID80187020 | |
Description | DSSTox provides a high quality public chemistry resource for supporting improved predictive toxicology. | |
Molecular Weight |
382.3 g/mol | |
Source | PubChem | |
URL | https://pubchem.ncbi.nlm.nih.gov | |
Description | Data deposited in or computed by PubChem | |
CAS No. |
33390-21-5 | |
Record name | Bikaverin | |
Source | ChemIDplus | |
URL | https://pubchem.ncbi.nlm.nih.gov/substance/?source=chemidplus&sourceid=0033390215 | |
Description | ChemIDplus is a free, web search system that provides access to the structure and nomenclature authority files used for the identification of chemical substances cited in National Library of Medicine (NLM) databases, including the TOXNET system. | |
Record name | Bikaverin | |
Source | DTP/NCI | |
URL | https://dtp.cancer.gov/dtpstandard/servlet/dwindex?searchtype=NSC&outputformat=html&searchlist=215139 | |
Description | The NCI Development Therapeutics Program (DTP) provides services and resources to the academic and private-sector research communities worldwide to facilitate the discovery and development of new cancer therapeutic agents. | |
Explanation | Unless otherwise indicated, all text within NCI products is free of copyright and may be reused without our permission. Credit the National Cancer Institute as the source. | |
Record name | Bikaverin | |
Source | EPA DSSTox | |
URL | https://comptox.epa.gov/dashboard/DTXSID80187020 | |
Description | DSSTox provides a high quality public chemistry resource for supporting improved predictive toxicology. | |
Record name | 6,11-dihydroxy-3,8-dimethoxy-1-methylbenzo[b]xanthene-7,10,12-trione | |
Source | European Chemicals Agency (ECHA) | |
URL | https://echa.europa.eu/information-on-chemicals | |
Description | The European Chemicals Agency (ECHA) is an agency of the European Union which is the driving force among regulatory authorities in implementing the EU's groundbreaking chemicals legislation for the benefit of human health and the environment as well as for innovation and competitiveness. | |
Explanation | Use of the information, documents and data from the ECHA website is subject to the terms and conditions of this Legal Notice, and subject to other binding limitations provided for under applicable law, the information, documents and data made available on the ECHA website may be reproduced, distributed and/or used, totally or in part, for non-commercial purposes provided that ECHA is acknowledged as the source: "Source: European Chemicals Agency, http://echa.europa.eu/". Such acknowledgement must be included in each copy of the material. ECHA permits and encourages organisations and individuals to create links to the ECHA website under the following cumulative conditions: Links can only be made to webpages that provide a link to the Legal Notice page. | |
Record name | BIKAVERIN | |
Source | FDA Global Substance Registration System (GSRS) | |
URL | https://gsrs.ncats.nih.gov/ginas/app/beta/substances/F1N6BX58PJ | |
Description | The FDA Global Substance Registration System (GSRS) enables the efficient and accurate exchange of information on what substances are in regulated products. Instead of relying on names, which vary across regulatory domains, countries, and regions, the GSRS knowledge base makes it possible for substances to be defined by standardized, scientific descriptions. | |
Explanation | Unless otherwise noted, the contents of the FDA website (www.fda.gov), both text and graphics, are not copyrighted. They are in the public domain and may be republished, reprinted and otherwise used freely by anyone without the need to obtain permission from FDA. Credit to the U.S. Food and Drug Administration as the source is appreciated but not required. | |
Retrosynthesis Analysis
AI-Powered Synthesis Planning: Our tool employs the Template_relevance Pistachio, Template_relevance Bkms_metabolic, Template_relevance Pistachio_ringbreaker, Template_relevance Reaxys, Template_relevance Reaxys_biocatalysis model, leveraging a vast database of chemical reactions to predict feasible synthetic routes.
One-Step Synthesis Focus: Specifically designed for one-step synthesis, it provides concise and direct routes for your target compounds, streamlining the synthesis process.
Accurate Predictions: Utilizing the extensive PISTACHIO, BKMS_METABOLIC, PISTACHIO_RINGBREAKER, REAXYS, REAXYS_BIOCATALYSIS database, our tool offers high-accuracy predictions, reflecting the latest in chemical research and data.
Strategy Settings
Precursor scoring | Relevance Heuristic |
---|---|
Min. plausibility | 0.01 |
Model | Template_relevance |
Template Set | Pistachio/Bkms_metabolic/Pistachio_ringbreaker/Reaxys/Reaxys_biocatalysis |
Top-N result to add to graph | 6 |
Feasible Synthetic Routes
Disclaimer and Information on In-Vitro Research Products
Please be aware that all articles and product information presented on BenchChem are intended solely for informational purposes. The products available for purchase on BenchChem are specifically designed for in-vitro studies, which are conducted outside of living organisms. In-vitro studies, derived from the Latin term "in glass," involve experiments performed in controlled laboratory settings using cells or tissues. It is important to note that these products are not categorized as medicines or drugs, and they have not received approval from the FDA for the prevention, treatment, or cure of any medical condition, ailment, or disease. We must emphasize that any form of bodily introduction of these products into humans or animals is strictly prohibited by law. It is essential to adhere to these guidelines to ensure compliance with legal and ethical standards in research and experimentation.