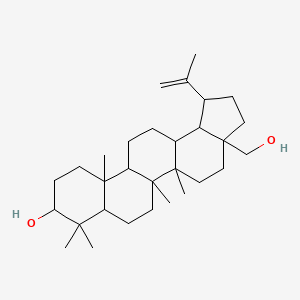
Betulin
Overview
Description
Betulin is a naturally occurring pentacyclic triterpene found in large quantities in the outer bark of birch trees (Betula species). It was first isolated in 1788 by the German-Russian chemist Johann Tobias Lowitz. This compound is known for its white crystalline appearance and is a significant component of birch bark, contributing to the tree’s white color. This compound has garnered attention due to its wide range of biological and pharmacological properties, including anti-inflammatory, antiviral, and anticancer activities .
Preparation Methods
Synthetic Routes and Reaction Conditions
Betulin can be synthesized through various methods, including vacuum sublimation after preliminary alkaline treatment, vacuum pyrolysis, supercritical extraction with carbon dioxide or mixtures of carbon dioxide with various solvents, and extraction with organic solvents . One notable method involves the use of microwave activation, which has been applied to the Kyrgyz birch (Betula kirghisorum), an endemic species in Kazakhstan .
Industrial Production Methods
Industrial production of this compound typically involves the extraction from birch bark, which is a byproduct of the woodworking industry. The extraction process can be optimized to obtain large quantities of this compound, making it a valuable resource for various applications .
Chemical Reactions Analysis
Types of Reactions
Betulin undergoes several types of chemical reactions, including oxidation, reduction, and substitution. These reactions are essential for modifying the compound to enhance its biological activity and solubility .
Common Reagents and Conditions
Common reagents used in the chemical reactions of this compound include sulfamic acid, ammonium sulfamate, and various solid catalysts such as Amberlyst 15® and Sibunit . These reagents facilitate the formation of sulfur derivatives of this compound, which have been examined using various analytical techniques .
Major Products Formed
The major products formed from the chemical reactions of this compound include betulinic acid, which is obtained through the oxidation of this compound. Betulinic acid is known for its potent anticancer properties and has been extensively studied for its therapeutic potential .
Scientific Research Applications
Betulin has a wide range of scientific research applications across various fields:
Mechanism of Action
Betulin exerts its effects through various molecular targets and pathways. It induces apoptosis in cancer cells by activating the intrinsic pathway, which involves the release of cytochrome c from mitochondria and the activation of caspases . This compound also modulates chronic inflammation by blocking the expression of proinflammatory cytokines via the NFκB and MAPKs pathways . Additionally, it reduces postprandial hyperglycemia by inhibiting α-amylase and α-glucosidase activity .
Comparison with Similar Compounds
Betulin is often compared with its oxidized derivative, betulinic acid. Both compounds share similar biological activities, but betulinic acid is more potent in inducing apoptosis in cancer cells . Other similar compounds include lupeol and oleanolic acid, which are also pentacyclic triterpenes with comparable pharmacological properties . this compound’s unique ability to be easily extracted from birch bark and its wide range of biological activities make it a valuable compound for various applications.
Conclusion
This compound is a versatile compound with significant potential in various scientific fields. Its wide range of biological activities, ease of extraction, and potential for chemical modification make it a valuable resource for research and industrial applications. As research continues, this compound and its derivatives may offer new therapeutic options for treating various diseases and improving human health.
Properties
IUPAC Name |
3a-(hydroxymethyl)-5a,5b,8,8,11a-pentamethyl-1-prop-1-en-2-yl-1,2,3,4,5,6,7,7a,9,10,11,11b,12,13,13a,13b-hexadecahydrocyclopenta[a]chrysen-9-ol | |
---|---|---|
Details | Computed by Lexichem TK 2.7.0 (PubChem release 2021.05.07) | |
Source | PubChem | |
URL | https://pubchem.ncbi.nlm.nih.gov | |
Description | Data deposited in or computed by PubChem | |
InChI |
InChI=1S/C30H50O2/c1-19(2)20-10-15-30(18-31)17-16-28(6)21(25(20)30)8-9-23-27(5)13-12-24(32)26(3,4)22(27)11-14-29(23,28)7/h20-25,31-32H,1,8-18H2,2-7H3 | |
Details | Computed by InChI 1.0.6 (PubChem release 2021.05.07) | |
Source | PubChem | |
URL | https://pubchem.ncbi.nlm.nih.gov | |
Description | Data deposited in or computed by PubChem | |
InChI Key |
FVWJYYTZTCVBKE-UHFFFAOYSA-N | |
Details | Computed by InChI 1.0.6 (PubChem release 2021.05.07) | |
Source | PubChem | |
URL | https://pubchem.ncbi.nlm.nih.gov | |
Description | Data deposited in or computed by PubChem | |
Canonical SMILES |
CC(=C)C1CCC2(C1C3CCC4C5(CCC(C(C5CCC4(C3(CC2)C)C)(C)C)O)C)CO | |
Details | Computed by OEChem 2.3.0 (PubChem release 2021.05.07) | |
Source | PubChem | |
URL | https://pubchem.ncbi.nlm.nih.gov | |
Description | Data deposited in or computed by PubChem | |
Molecular Formula |
C30H50O2 | |
Details | Computed by PubChem 2.1 (PubChem release 2021.05.07) | |
Source | PubChem | |
URL | https://pubchem.ncbi.nlm.nih.gov | |
Description | Data deposited in or computed by PubChem | |
Molecular Weight |
442.7 g/mol | |
Details | Computed by PubChem 2.1 (PubChem release 2021.05.07) | |
Source | PubChem | |
URL | https://pubchem.ncbi.nlm.nih.gov | |
Description | Data deposited in or computed by PubChem | |
CAS No. |
473-98-3 | |
Record name | Betulin | |
Source | DTP/NCI | |
URL | https://dtp.cancer.gov/dtpstandard/servlet/dwindex?searchtype=NSC&outputformat=html&searchlist=4644 | |
Description | The NCI Development Therapeutics Program (DTP) provides services and resources to the academic and private-sector research communities worldwide to facilitate the discovery and development of new cancer therapeutic agents. | |
Explanation | Unless otherwise indicated, all text within NCI products is free of copyright and may be reused without our permission. Credit the National Cancer Institute as the source. | |
Retrosynthesis Analysis
AI-Powered Synthesis Planning: Our tool employs the Template_relevance Pistachio, Template_relevance Bkms_metabolic, Template_relevance Pistachio_ringbreaker, Template_relevance Reaxys, Template_relevance Reaxys_biocatalysis model, leveraging a vast database of chemical reactions to predict feasible synthetic routes.
One-Step Synthesis Focus: Specifically designed for one-step synthesis, it provides concise and direct routes for your target compounds, streamlining the synthesis process.
Accurate Predictions: Utilizing the extensive PISTACHIO, BKMS_METABOLIC, PISTACHIO_RINGBREAKER, REAXYS, REAXYS_BIOCATALYSIS database, our tool offers high-accuracy predictions, reflecting the latest in chemical research and data.
Strategy Settings
Precursor scoring | Relevance Heuristic |
---|---|
Min. plausibility | 0.01 |
Model | Template_relevance |
Template Set | Pistachio/Bkms_metabolic/Pistachio_ringbreaker/Reaxys/Reaxys_biocatalysis |
Top-N result to add to graph | 6 |
Feasible Synthetic Routes
Disclaimer and Information on In-Vitro Research Products
Please be aware that all articles and product information presented on BenchChem are intended solely for informational purposes. The products available for purchase on BenchChem are specifically designed for in-vitro studies, which are conducted outside of living organisms. In-vitro studies, derived from the Latin term "in glass," involve experiments performed in controlled laboratory settings using cells or tissues. It is important to note that these products are not categorized as medicines or drugs, and they have not received approval from the FDA for the prevention, treatment, or cure of any medical condition, ailment, or disease. We must emphasize that any form of bodily introduction of these products into humans or animals is strictly prohibited by law. It is essential to adhere to these guidelines to ensure compliance with legal and ethical standards in research and experimentation.