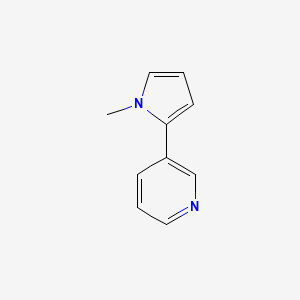
Nicotyrine
Overview
Description
Nicotyrine is a lesser-known and minor tobacco alkaloid . It inhibits the metabolism of nicotine through CYP2A6 enzyme inhibition . It is formed by the gradual oxidation of nicotine in e-liquids and causes delayed nicotine clearance and attenuated withdrawal symptoms . It also has insecticidal properties like nicotine .
Synthesis Analysis
Nicotyrine can be produced readily from nicotine by catalytic dehydrogenation . It can also be produced from tobacco biomass by catalytic pyrolysis . There is significant regulatory and economic need to distinguish analytically between tobacco-derived nicotine (TDN) and synthetic nicotine (SyN) in commercial products .Molecular Structure Analysis
The molecular formula of Nicotyrine is C10H10N2 . The IUPAC name is 3-(1-methylpyrrol-2-yl)pyridine . The molecular weight is 158.20 g/mol .Chemical Reactions Analysis
Nicotyrine is formed by the gradual oxidation of nicotine in e-liquids . Techniques to accomplish this include screening of nicotine samples for impurities such as nicotine degradants and metals, chiral analysis of nicotine and nornicotine, and radiocarbon analysis .Physical And Chemical Properties Analysis
Nicotyrine is a member of pyridines . It is a natural product found in Nicotiana tabacum .Scientific Research Applications
Pharmacological Inhibitor
Nicotyrine acts as a potent inhibitor of cytochrome P450 enzymes, particularly CYP2A13 and CYP2A6, which are involved in nicotine metabolism. This inhibition can lead to delayed nicotine clearance and attenuated withdrawal symptoms, which may have implications for smoking cessation therapies .
Insecticidal Properties
Like nicotine, Nicotyrine has been found to possess insecticidal properties. Certain derivatives of Nicotyrine have been synthesized specifically to exploit this characteristic for pest control applications .
E-Cigarette Aerosol Analysis
Studies have analyzed e-cigarette aerosols on-line using a Thermal Desorption Aerosol Gas Chromatograph to understand the formation of Nicotyrine in e-liquids and its potential impact on nicotine metabolism .
Impact on Serum Nicotine Levels
Research suggests that when inhaled together with nicotine, Nicotyrine can reversibly inhibit nicotine metabolism in the airways by CYP2A13, leading to measurable serum nicotine levels in e-cigarette users .
E-Liquid Aging and Nicotyrine Formation
An aging experiment determined the nicotyrine/nicotine ratio (NNR) in e-liquids and aerosols as a function of time since initial exposure to air and storage conditions, providing insights into the stability and chemical composition of e-liquids over time .
Mechanism of Action
Target of Action
Nicotyrine, a minor tobacco alkaloid, primarily targets the cytochrome P450 enzymes CYP2A6 and CYP2A13 . These enzymes play a significant role in the metabolism of nicotine .
Mode of Action
Nicotyrine acts as an inhibitor of its primary targets, CYP2A6 and CYP2A13 . By inhibiting these enzymes, Nicotyrine affects the metabolism of nicotine, leading to delayed nicotine clearance and attenuated withdrawal symptoms .
Biochemical Pathways
The biochemical pathways affected by Nicotyrine are primarily those involved in the metabolism of nicotine . Nicotyrine inhibits the CYP2A6 and CYP2A13 enzymes, which play a key role in nicotine metabolism . This inhibition results in a decrease in the rate of nicotine metabolism, leading to prolonged nicotine presence in the body .
Pharmacokinetics
The pharmacokinetics of Nicotyrine are closely tied to its role in inhibiting nicotine metabolism . By inhibiting the CYP2A6 and CYP2A13 enzymes, Nicotyrine affects the Absorption, Distribution, Metabolism, and Excretion (ADME) properties of nicotine . This results in delayed nicotine clearance and prolonged presence of nicotine in the body .
Result of Action
The primary molecular and cellular effect of Nicotyrine’s action is the inhibition of nicotine metabolism . This leads to a delay in nicotine clearance and attenuated withdrawal symptoms . On a cellular level, this can result in prolonged exposure to nicotine, potentially affecting various cellular processes and responses .
Action Environment
Environmental factors can influence the action, efficacy, and stability of Nicotyrine. For instance, diet, age, sex, use of certain medications, and smoking itself can influence nicotine metabolism, and by extension, the action of Nicotyrine . Furthermore, racial and ethnic differences, likely influenced by both genetic and environmental factors, have been observed in nicotine metabolism .
Future Directions
There is significant regulatory and economic need to distinguish analytically between tobacco-derived nicotine (TDN) and synthetic nicotine (SyN) in commercial products . This regulatory difference provides an economic incentive to use or claim the use of SyN to remain on the market without submitting a Premarket Tobacco Product Application .
properties
IUPAC Name |
3-(1-methylpyrrol-2-yl)pyridine | |
---|---|---|
Source | PubChem | |
URL | https://pubchem.ncbi.nlm.nih.gov | |
Description | Data deposited in or computed by PubChem | |
InChI |
InChI=1S/C10H10N2/c1-12-7-3-5-10(12)9-4-2-6-11-8-9/h2-8H,1H3 | |
Source | PubChem | |
URL | https://pubchem.ncbi.nlm.nih.gov | |
Description | Data deposited in or computed by PubChem | |
InChI Key |
RYFOJXFXERAMLS-UHFFFAOYSA-N | |
Source | PubChem | |
URL | https://pubchem.ncbi.nlm.nih.gov | |
Description | Data deposited in or computed by PubChem | |
Canonical SMILES |
CN1C=CC=C1C2=CN=CC=C2 | |
Source | PubChem | |
URL | https://pubchem.ncbi.nlm.nih.gov | |
Description | Data deposited in or computed by PubChem | |
Molecular Formula |
C10H10N2 | |
Source | PubChem | |
URL | https://pubchem.ncbi.nlm.nih.gov | |
Description | Data deposited in or computed by PubChem | |
DSSTOX Substance ID |
DTXSID3075048 | |
Record name | Pyridine, 3-(1-methyl-1H-pyrrol-2-yl)- | |
Source | EPA DSSTox | |
URL | https://comptox.epa.gov/dashboard/DTXSID3075048 | |
Description | DSSTox provides a high quality public chemistry resource for supporting improved predictive toxicology. | |
Molecular Weight |
158.20 g/mol | |
Source | PubChem | |
URL | https://pubchem.ncbi.nlm.nih.gov | |
Description | Data deposited in or computed by PubChem | |
Product Name |
Nicotyrine | |
CAS RN |
487-19-4 | |
Record name | Nicotyrine | |
Source | CAS Common Chemistry | |
URL | https://commonchemistry.cas.org/detail?cas_rn=487-19-4 | |
Description | CAS Common Chemistry is an open community resource for accessing chemical information. Nearly 500,000 chemical substances from CAS REGISTRY cover areas of community interest, including common and frequently regulated chemicals, and those relevant to high school and undergraduate chemistry classes. This chemical information, curated by our expert scientists, is provided in alignment with our mission as a division of the American Chemical Society. | |
Explanation | The data from CAS Common Chemistry is provided under a CC-BY-NC 4.0 license, unless otherwise stated. | |
Record name | alpha-Nicotyrine | |
Source | ChemIDplus | |
URL | https://pubchem.ncbi.nlm.nih.gov/substance/?source=chemidplus&sourceid=0000487194 | |
Description | ChemIDplus is a free, web search system that provides access to the structure and nomenclature authority files used for the identification of chemical substances cited in National Library of Medicine (NLM) databases, including the TOXNET system. | |
Record name | Nicotyrine | |
Source | DTP/NCI | |
URL | https://dtp.cancer.gov/dtpstandard/servlet/dwindex?searchtype=NSC&outputformat=html&searchlist=407276 | |
Description | The NCI Development Therapeutics Program (DTP) provides services and resources to the academic and private-sector research communities worldwide to facilitate the discovery and development of new cancer therapeutic agents. | |
Explanation | Unless otherwise indicated, all text within NCI products is free of copyright and may be reused without our permission. Credit the National Cancer Institute as the source. | |
Record name | Nicotyrine | |
Source | DTP/NCI | |
URL | https://dtp.cancer.gov/dtpstandard/servlet/dwindex?searchtype=NSC&outputformat=html&searchlist=127943 | |
Description | The NCI Development Therapeutics Program (DTP) provides services and resources to the academic and private-sector research communities worldwide to facilitate the discovery and development of new cancer therapeutic agents. | |
Explanation | Unless otherwise indicated, all text within NCI products is free of copyright and may be reused without our permission. Credit the National Cancer Institute as the source. | |
Record name | Pyridine, 3-(1-methyl-1H-pyrrol-2-yl)- | |
Source | EPA DSSTox | |
URL | https://comptox.epa.gov/dashboard/DTXSID3075048 | |
Description | DSSTox provides a high quality public chemistry resource for supporting improved predictive toxicology. | |
Record name | NICOTYRINE | |
Source | FDA Global Substance Registration System (GSRS) | |
URL | https://gsrs.ncats.nih.gov/ginas/app/beta/substances/XN4R1LH79Y | |
Description | The FDA Global Substance Registration System (GSRS) enables the efficient and accurate exchange of information on what substances are in regulated products. Instead of relying on names, which vary across regulatory domains, countries, and regions, the GSRS knowledge base makes it possible for substances to be defined by standardized, scientific descriptions. | |
Explanation | Unless otherwise noted, the contents of the FDA website (www.fda.gov), both text and graphics, are not copyrighted. They are in the public domain and may be republished, reprinted and otherwise used freely by anyone without the need to obtain permission from FDA. Credit to the U.S. Food and Drug Administration as the source is appreciated but not required. | |
Retrosynthesis Analysis
AI-Powered Synthesis Planning: Our tool employs the Template_relevance Pistachio, Template_relevance Bkms_metabolic, Template_relevance Pistachio_ringbreaker, Template_relevance Reaxys, Template_relevance Reaxys_biocatalysis model, leveraging a vast database of chemical reactions to predict feasible synthetic routes.
One-Step Synthesis Focus: Specifically designed for one-step synthesis, it provides concise and direct routes for your target compounds, streamlining the synthesis process.
Accurate Predictions: Utilizing the extensive PISTACHIO, BKMS_METABOLIC, PISTACHIO_RINGBREAKER, REAXYS, REAXYS_BIOCATALYSIS database, our tool offers high-accuracy predictions, reflecting the latest in chemical research and data.
Strategy Settings
Precursor scoring | Relevance Heuristic |
---|---|
Min. plausibility | 0.01 |
Model | Template_relevance |
Template Set | Pistachio/Bkms_metabolic/Pistachio_ringbreaker/Reaxys/Reaxys_biocatalysis |
Top-N result to add to graph | 6 |
Feasible Synthetic Routes
Disclaimer and Information on In-Vitro Research Products
Please be aware that all articles and product information presented on BenchChem are intended solely for informational purposes. The products available for purchase on BenchChem are specifically designed for in-vitro studies, which are conducted outside of living organisms. In-vitro studies, derived from the Latin term "in glass," involve experiments performed in controlled laboratory settings using cells or tissues. It is important to note that these products are not categorized as medicines or drugs, and they have not received approval from the FDA for the prevention, treatment, or cure of any medical condition, ailment, or disease. We must emphasize that any form of bodily introduction of these products into humans or animals is strictly prohibited by law. It is essential to adhere to these guidelines to ensure compliance with legal and ethical standards in research and experimentation.