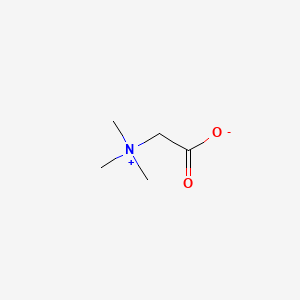
Betaine
Overview
Description
. It is a zwitterionic quaternary ammonium compound derived from the amino acid glycine. Betaine is widely distributed in animals, plants, and microorganisms, and it plays a crucial role as an osmoprotectant and methyl group donor .
Mechanism of Action
Target of Action
Betaine, also known as trimethylglycine, is a naturally occurring choline derivative . It primarily targets homocysteine , a non-proteinogenic α-amino acid . This compound acts as a methyl group donor in the conversion of homocysteine to methionine . This reaction is catalyzed by the enzyme This compound-homocysteine methyltransferase (BHMT) , which is mainly expressed in the liver and kidneys .
Mode of Action
This compound donates a methyl group to homocysteine, forming N,N-dimethylglycine and L-methionine . Methionine is then converted to S-adenosylmethionine (SAM), which serves as a methyl donor in DNA methylation and many anabolic pathways of phospholipids, hormones, and proteins .
Biochemical Pathways
This compound is involved in the methionine cycle , where it plays a pivotal role in numerous pathways . It participates in the remethylation of homocysteine to methionine, which is then converted to SAM . SAM is a universal methyl donor, contributing to the methylation of a wide range of substrates, including DNA, proteins, and lipids .
Pharmacokinetics
This compound can be obtained from dietary sources or synthesized endogenously from choline . After ingestion, this compound is absorbed from the duodenal enterocytes into circulation . It is then transported to the liver and kidneys, where it is catabolized by a series of enzyme-catalyzed reactions, predominantly in the mitochondria . The removal of this compound from the body is primarily by metabolism, with minimal urinary excretion, even after high amounts of this compound consumption .
Result of Action
The primary result of this compound’s action is the reduction of plasma homocysteine levels . Elevated homocysteine levels are associated with an increased risk of cardiovascular diseases . Therefore, this compound’s ability to lower homocysteine levels contributes to its cardioprotective effects . Additionally, this compound has been shown to have potential benefits for fighting heart disease, improving body composition, and promoting muscle gain and fat loss due to its abilities to promote protein synthesis in the body .
Action Environment
This compound’s action is influenced by environmental factors such as osmotic stress, temperature stress, and drought . As an osmoprotectant, this compound helps to maintain normal cell volume under osmotic stress . It can accumulate to molar concentrations, providing protection against protein denaturation . Therefore, the efficiency of this compound’s transport systems usually increases at high osmolalities, with transcriptional and post-transcriptional adjustments occurring in response to changes in osmotic stress .
Biochemical Analysis
Biochemical Properties
Betaine plays a pivotal role in numerous pathways, including the methionine cycle . Due to its ability to donate a methyl group, it interacts with enzymes, proteins, and other biomolecules . For instance, this compound donates a methyl group to homocysteine to form N,N-dimethylglycine and L-methionine via the enzyme this compound-homocysteine methyltransferase (BHMT) .
Cellular Effects
This compound has significant effects on various types of cells and cellular processes. It influences cell function, including impact on cell signaling pathways, gene expression, and cellular metabolism . A this compound-deficient diet can disturb several cellular processes .
Molecular Mechanism
This compound exerts its effects at the molecular level, including binding interactions with biomolecules, enzyme inhibition or activation, and changes in gene expression . It is catabolized by a series of enzyme-catalyzed reactions, predominantly in the mitochondria, and participates in the methionine cycle .
Temporal Effects in Laboratory Settings
The effects of this compound change over time in laboratory settings. Information on the product’s stability, degradation, and any long-term effects on cellular function observed in in vitro or in vivo studies is still being researched .
Dosage Effects in Animal Models
The effects of this compound vary with different dosages in animal models. This includes any threshold effects observed in these studies, as well as any toxic or adverse effects at high doses .
Metabolic Pathways
This compound is involved in the methionine cycle . It interacts with enzymes or cofactors, and it affects metabolic flux or metabolite levels .
Transport and Distribution
This compound is absorbed from the duodenal enterocytes into circulation and maintained between 20 and 70 µmol L −1 with a median plasma concentration of 27.8 µmol L −1 . It is carried to the liver and kidneys where it is catabolized .
Subcellular Localization
The subcellular localization of this compound and any effects on its activity or function are still being researched . This could include any targeting signals or post-translational modifications that direct it to specific compartments or organelles .
Preparation Methods
Synthetic Routes and Reaction Conditions: Betaine can be synthesized through the oxidation of choline. The process involves two main steps: the oxidation of choline to this compound aldehyde, followed by the oxidation of this compound aldehyde to this compound . The reaction conditions typically involve the use of oxidizing agents such as hydrogen peroxide or potassium permanganate.
Industrial Production Methods: Industrial production of this compound often involves extraction from natural sources such as sugar beets. The extraction process includes steps like accelerated solvent extraction coupled with solid-phase extraction . This method ensures high recovery rates and purity of the compound.
Chemical Reactions Analysis
Types of Reactions: Betaine undergoes various chemical reactions, including:
Oxidation: this compound can be oxidized to form dimethylglycine and formaldehyde.
Reduction: this compound can be reduced to form trimethylamine and acetic acid.
Substitution: this compound can participate in substitution reactions, where the trimethylammonium group is replaced by other functional groups.
Common Reagents and Conditions:
Oxidizing Agents: Hydrogen peroxide, potassium permanganate.
Reducing Agents: Sodium borohydride, lithium aluminum hydride.
Substitution Reagents: Alkyl halides, acyl chlorides.
Major Products Formed:
Oxidation Products: Dimethylglycine, formaldehyde.
Reduction Products: Trimethylamine, acetic acid.
Substitution Products: Various substituted ammonium compounds.
Scientific Research Applications
Betaine has a wide range of applications in scientific research, including:
Biology: this compound plays a role in cellular osmoregulation and protects cells from osmotic stress.
Medicine: this compound is used to treat homocystinuria, a genetic disorder that leads to the accumulation of homocysteine in the blood.
Industry: this compound is used as a feed additive in animal nutrition to improve growth performance and feed efficiency.
Comparison with Similar Compounds
Betaine is often compared with other similar compounds, such as:
Dimethylglycine: Like this compound, dimethylglycine is a methyl donor but lacks the quaternary ammonium group.
Choline: Choline is a precursor to this compound and also serves as a methyl donor and osmoprotectant.
Uniqueness of this compound: this compound’s unique combination of osmoprotective and methyl donor properties makes it distinct from other similar compounds. Its ability to stabilize proteins and enzymes under stress conditions, along with its role in methylation reactions, highlights its versatility and importance in various biological and industrial applications .
Properties
IUPAC Name |
2-(trimethylazaniumyl)acetate | |
---|---|---|
Source | PubChem | |
URL | https://pubchem.ncbi.nlm.nih.gov | |
Description | Data deposited in or computed by PubChem | |
InChI |
InChI=1S/C5H11NO2/c1-6(2,3)4-5(7)8/h4H2,1-3H3 | |
Source | PubChem | |
URL | https://pubchem.ncbi.nlm.nih.gov | |
Description | Data deposited in or computed by PubChem | |
InChI Key |
KWIUHFFTVRNATP-UHFFFAOYSA-N | |
Source | PubChem | |
URL | https://pubchem.ncbi.nlm.nih.gov | |
Description | Data deposited in or computed by PubChem | |
Canonical SMILES |
C[N+](C)(C)CC(=O)[O-] | |
Source | PubChem | |
URL | https://pubchem.ncbi.nlm.nih.gov | |
Description | Data deposited in or computed by PubChem | |
Molecular Formula |
C5H11NO2 | |
Source | PubChem | |
URL | https://pubchem.ncbi.nlm.nih.gov | |
Description | Data deposited in or computed by PubChem | |
Related CAS |
590-46-5 (hydrochloride) | |
Record name | Betaine | |
Source | ChemIDplus | |
URL | https://pubchem.ncbi.nlm.nih.gov/substance/?source=chemidplus&sourceid=0000107437 | |
Description | ChemIDplus is a free, web search system that provides access to the structure and nomenclature authority files used for the identification of chemical substances cited in National Library of Medicine (NLM) databases, including the TOXNET system. | |
DSSTOX Substance ID |
DTXSID8022666 | |
Record name | Betaine | |
Source | EPA DSSTox | |
URL | https://comptox.epa.gov/dashboard/DTXSID8022666 | |
Description | DSSTox provides a high quality public chemistry resource for supporting improved predictive toxicology. | |
Molecular Weight |
117.15 g/mol | |
Source | PubChem | |
URL | https://pubchem.ncbi.nlm.nih.gov | |
Description | Data deposited in or computed by PubChem | |
Physical Description |
Liquid, Deliquescent solid; [Merck Index] Powder; [Alfa Aesar MSDS], Free-flowing, white crystals; Savoury | |
Record name | Methanaminium, 1-carboxy-N,N,N-trimethyl-, inner salt | |
Source | EPA Chemicals under the TSCA | |
URL | https://www.epa.gov/chemicals-under-tsca | |
Description | EPA Chemicals under the Toxic Substances Control Act (TSCA) collection contains information on chemicals and their regulations under TSCA, including non-confidential content from the TSCA Chemical Substance Inventory and Chemical Data Reporting. | |
Record name | Betaine | |
Source | Haz-Map, Information on Hazardous Chemicals and Occupational Diseases | |
URL | https://haz-map.com/Agents/3916 | |
Description | Haz-Map® is an occupational health database designed for health and safety professionals and for consumers seeking information about the adverse effects of workplace exposures to chemical and biological agents. | |
Explanation | Copyright (c) 2022 Haz-Map(R). All rights reserved. Unless otherwise indicated, all materials from Haz-Map are copyrighted by Haz-Map(R). No part of these materials, either text or image may be used for any purpose other than for personal use. Therefore, reproduction, modification, storage in a retrieval system or retransmission, in any form or by any means, electronic, mechanical or otherwise, for reasons other than personal use, is strictly prohibited without prior written permission. | |
Record name | Betaine | |
Source | Joint FAO/WHO Expert Committee on Food Additives (JECFA) | |
URL | https://www.fao.org/food/food-safety-quality/scientific-advice/jecfa/jecfa-flav/details/en/c/2283/ | |
Description | The flavoring agent databse provides the most recent specifications for flavorings evaluated by JECFA. | |
Explanation | Permission from WHO is not required for the use of WHO materials issued under the Creative Commons Attribution-NonCommercial-ShareAlike 3.0 Intergovernmental Organization (CC BY-NC-SA 3.0 IGO) licence. | |
Solubility |
Soluble, Solubility (g/100 g solvent): methanol, 55; ethanol, 8.7. Sparingly soluble in ether., Slightly soluble in chloroform, In water, 6.11X10+5 mg/L at 19 °C, Very soluble, Soluble (in ethanol) | |
Record name | Betaine | |
Source | DrugBank | |
URL | https://www.drugbank.ca/drugs/DB06756 | |
Description | The DrugBank database is a unique bioinformatics and cheminformatics resource that combines detailed drug (i.e. chemical, pharmacological and pharmaceutical) data with comprehensive drug target (i.e. sequence, structure, and pathway) information. | |
Explanation | Creative Common's Attribution-NonCommercial 4.0 International License (http://creativecommons.org/licenses/by-nc/4.0/legalcode) | |
Record name | BETAINE | |
Source | Hazardous Substances Data Bank (HSDB) | |
URL | https://pubchem.ncbi.nlm.nih.gov/source/hsdb/7467 | |
Description | The Hazardous Substances Data Bank (HSDB) is a toxicology database that focuses on the toxicology of potentially hazardous chemicals. It provides information on human exposure, industrial hygiene, emergency handling procedures, environmental fate, regulatory requirements, nanomaterials, and related areas. The information in HSDB has been assessed by a Scientific Review Panel. | |
Record name | Betaine | |
Source | Joint FAO/WHO Expert Committee on Food Additives (JECFA) | |
URL | https://www.fao.org/food/food-safety-quality/scientific-advice/jecfa/jecfa-flav/details/en/c/2283/ | |
Description | The flavoring agent databse provides the most recent specifications for flavorings evaluated by JECFA. | |
Explanation | Permission from WHO is not required for the use of WHO materials issued under the Creative Commons Attribution-NonCommercial-ShareAlike 3.0 Intergovernmental Organization (CC BY-NC-SA 3.0 IGO) licence. | |
Vapor Pressure |
0.00000053 [mmHg] | |
Record name | Betaine | |
Source | Haz-Map, Information on Hazardous Chemicals and Occupational Diseases | |
URL | https://haz-map.com/Agents/3916 | |
Description | Haz-Map® is an occupational health database designed for health and safety professionals and for consumers seeking information about the adverse effects of workplace exposures to chemical and biological agents. | |
Explanation | Copyright (c) 2022 Haz-Map(R). All rights reserved. Unless otherwise indicated, all materials from Haz-Map are copyrighted by Haz-Map(R). No part of these materials, either text or image may be used for any purpose other than for personal use. Therefore, reproduction, modification, storage in a retrieval system or retransmission, in any form or by any means, electronic, mechanical or otherwise, for reasons other than personal use, is strictly prohibited without prior written permission. | |
Mechanism of Action |
Homocystinuria is a hereditary disorder characterized by high levels of the amino acid homocysteine. This condition can be caused by deficiencies or defects in cystathionine beta-synthase (CBS), 5,10-methylenetetrahydrofolate reductase (MTHFR), and cobalamin cofactor metabolism (cbl). CBS converts homocysteine to cystathionine, and a deficiency in this enzyme can lead to an accumulation of homocysteine. MTHFR is responsible for producing 5-methyltetrahydrofolate, a methyl donor that participates in the conversion of homocysteine back to methionine; therefore, an MTHFR deficiency can also lead to homocystinuria. Additionally, a defect in cobalamin (vitamin B12) cofactor metabolism can lead to homocystinuria, since a metabolite of cobalamin (methylcobalamin) promotes the conversion of homocysteine to methionine. Betaine transfers a methyl group via the enzyme betaine homocysteine methyl transferase (BHMT), converting homocysteine back into methionine and dimethylglycine (DMG). In patients with homocystinuria, betaine reduces homocysteine levels and improves health outcomes., Betaine acts as metabolic intermediate in transmethylating processes (creatine and methionine synthesis)., Betaine acts as a methyl group donor in the remethylation of homocysteine to methionine in patients with homocystinuria. This reduces toxic concentrations of homocysteine, usually to 20 to 30% or less of pretreatment concentrations., Betaine or trimethylglycine is a quarternary ammonium compound that was first discovered in the juice of sugar beets (Beta vulgaris). Betaine is a metabolite of choline ... and is a substrate in one of the two recycling pathways that convert homocysteine to L-methionine. The other and principal recycling reaction is catalyzed by the enzyme methionine synthase and uses methylcobalamin as a cofactor and 5-methyltetrahydrofolate as a cosubstrate., Betaine-homocysteine methyltransferase (BHMT) is a zinc metalloenzyme which catalyzes the transfer of a methyl group from betaine to homocysteine in the formation of methionine. BHMT is found in the liver and kidneys and may also exist in brain tissue. Betaine acts to lower homocysteine levels in some with primary hyperhomocysteinemia/homocystinuria via this enzyme. | |
Record name | Betaine | |
Source | DrugBank | |
URL | https://www.drugbank.ca/drugs/DB06756 | |
Description | The DrugBank database is a unique bioinformatics and cheminformatics resource that combines detailed drug (i.e. chemical, pharmacological and pharmaceutical) data with comprehensive drug target (i.e. sequence, structure, and pathway) information. | |
Explanation | Creative Common's Attribution-NonCommercial 4.0 International License (http://creativecommons.org/licenses/by-nc/4.0/legalcode) | |
Record name | BETAINE | |
Source | Hazardous Substances Data Bank (HSDB) | |
URL | https://pubchem.ncbi.nlm.nih.gov/source/hsdb/7467 | |
Description | The Hazardous Substances Data Bank (HSDB) is a toxicology database that focuses on the toxicology of potentially hazardous chemicals. It provides information on human exposure, industrial hygiene, emergency handling procedures, environmental fate, regulatory requirements, nanomaterials, and related areas. The information in HSDB has been assessed by a Scientific Review Panel. | |
Color/Form |
Deliquescent scales or prisms | |
CAS No. |
107-43-7 | |
Record name | Betaine | |
Source | CAS Common Chemistry | |
URL | https://commonchemistry.cas.org/detail?cas_rn=107-43-7 | |
Description | CAS Common Chemistry is an open community resource for accessing chemical information. Nearly 500,000 chemical substances from CAS REGISTRY cover areas of community interest, including common and frequently regulated chemicals, and those relevant to high school and undergraduate chemistry classes. This chemical information, curated by our expert scientists, is provided in alignment with our mission as a division of the American Chemical Society. | |
Explanation | The data from CAS Common Chemistry is provided under a CC-BY-NC 4.0 license, unless otherwise stated. | |
Record name | Betaine | |
Source | ChemIDplus | |
URL | https://pubchem.ncbi.nlm.nih.gov/substance/?source=chemidplus&sourceid=0000107437 | |
Description | ChemIDplus is a free, web search system that provides access to the structure and nomenclature authority files used for the identification of chemical substances cited in National Library of Medicine (NLM) databases, including the TOXNET system. | |
Record name | Betaine | |
Source | DrugBank | |
URL | https://www.drugbank.ca/drugs/DB06756 | |
Description | The DrugBank database is a unique bioinformatics and cheminformatics resource that combines detailed drug (i.e. chemical, pharmacological and pharmaceutical) data with comprehensive drug target (i.e. sequence, structure, and pathway) information. | |
Explanation | Creative Common's Attribution-NonCommercial 4.0 International License (http://creativecommons.org/licenses/by-nc/4.0/legalcode) | |
Record name | betaine | |
Source | DTP/NCI | |
URL | https://dtp.cancer.gov/dtpstandard/servlet/dwindex?searchtype=NSC&outputformat=html&searchlist=166511 | |
Description | The NCI Development Therapeutics Program (DTP) provides services and resources to the academic and private-sector research communities worldwide to facilitate the discovery and development of new cancer therapeutic agents. | |
Explanation | Unless otherwise indicated, all text within NCI products is free of copyright and may be reused without our permission. Credit the National Cancer Institute as the source. | |
Record name | Methanaminium, 1-carboxy-N,N,N-trimethyl-, inner salt | |
Source | EPA Chemicals under the TSCA | |
URL | https://www.epa.gov/chemicals-under-tsca | |
Description | EPA Chemicals under the Toxic Substances Control Act (TSCA) collection contains information on chemicals and their regulations under TSCA, including non-confidential content from the TSCA Chemical Substance Inventory and Chemical Data Reporting. | |
Record name | Betaine | |
Source | EPA DSSTox | |
URL | https://comptox.epa.gov/dashboard/DTXSID8022666 | |
Description | DSSTox provides a high quality public chemistry resource for supporting improved predictive toxicology. | |
Record name | Betaine | |
Source | European Chemicals Agency (ECHA) | |
URL | https://echa.europa.eu/substance-information/-/substanceinfo/100.003.174 | |
Description | The European Chemicals Agency (ECHA) is an agency of the European Union which is the driving force among regulatory authorities in implementing the EU's groundbreaking chemicals legislation for the benefit of human health and the environment as well as for innovation and competitiveness. | |
Explanation | Use of the information, documents and data from the ECHA website is subject to the terms and conditions of this Legal Notice, and subject to other binding limitations provided for under applicable law, the information, documents and data made available on the ECHA website may be reproduced, distributed and/or used, totally or in part, for non-commercial purposes provided that ECHA is acknowledged as the source: "Source: European Chemicals Agency, http://echa.europa.eu/". Such acknowledgement must be included in each copy of the material. ECHA permits and encourages organisations and individuals to create links to the ECHA website under the following cumulative conditions: Links can only be made to webpages that provide a link to the Legal Notice page. | |
Record name | BETAINE | |
Source | FDA Global Substance Registration System (GSRS) | |
URL | https://gsrs.ncats.nih.gov/ginas/app/beta/substances/3SCV180C9W | |
Description | The FDA Global Substance Registration System (GSRS) enables the efficient and accurate exchange of information on what substances are in regulated products. Instead of relying on names, which vary across regulatory domains, countries, and regions, the GSRS knowledge base makes it possible for substances to be defined by standardized, scientific descriptions. | |
Explanation | Unless otherwise noted, the contents of the FDA website (www.fda.gov), both text and graphics, are not copyrighted. They are in the public domain and may be republished, reprinted and otherwise used freely by anyone without the need to obtain permission from FDA. Credit to the U.S. Food and Drug Administration as the source is appreciated but not required. | |
Record name | BETAINE | |
Source | Hazardous Substances Data Bank (HSDB) | |
URL | https://pubchem.ncbi.nlm.nih.gov/source/hsdb/7467 | |
Description | The Hazardous Substances Data Bank (HSDB) is a toxicology database that focuses on the toxicology of potentially hazardous chemicals. It provides information on human exposure, industrial hygiene, emergency handling procedures, environmental fate, regulatory requirements, nanomaterials, and related areas. The information in HSDB has been assessed by a Scientific Review Panel. | |
Melting Point |
301-305°C, Decomposes around 293 °C | |
Record name | Betaine | |
Source | DrugBank | |
URL | https://www.drugbank.ca/drugs/DB06756 | |
Description | The DrugBank database is a unique bioinformatics and cheminformatics resource that combines detailed drug (i.e. chemical, pharmacological and pharmaceutical) data with comprehensive drug target (i.e. sequence, structure, and pathway) information. | |
Explanation | Creative Common's Attribution-NonCommercial 4.0 International License (http://creativecommons.org/licenses/by-nc/4.0/legalcode) | |
Record name | BETAINE | |
Source | Hazardous Substances Data Bank (HSDB) | |
URL | https://pubchem.ncbi.nlm.nih.gov/source/hsdb/7467 | |
Description | The Hazardous Substances Data Bank (HSDB) is a toxicology database that focuses on the toxicology of potentially hazardous chemicals. It provides information on human exposure, industrial hygiene, emergency handling procedures, environmental fate, regulatory requirements, nanomaterials, and related areas. The information in HSDB has been assessed by a Scientific Review Panel. | |
Retrosynthesis Analysis
AI-Powered Synthesis Planning: Our tool employs the Template_relevance Pistachio, Template_relevance Bkms_metabolic, Template_relevance Pistachio_ringbreaker, Template_relevance Reaxys, Template_relevance Reaxys_biocatalysis model, leveraging a vast database of chemical reactions to predict feasible synthetic routes.
One-Step Synthesis Focus: Specifically designed for one-step synthesis, it provides concise and direct routes for your target compounds, streamlining the synthesis process.
Accurate Predictions: Utilizing the extensive PISTACHIO, BKMS_METABOLIC, PISTACHIO_RINGBREAKER, REAXYS, REAXYS_BIOCATALYSIS database, our tool offers high-accuracy predictions, reflecting the latest in chemical research and data.
Strategy Settings
Precursor scoring | Relevance Heuristic |
---|---|
Min. plausibility | 0.01 |
Model | Template_relevance |
Template Set | Pistachio/Bkms_metabolic/Pistachio_ringbreaker/Reaxys/Reaxys_biocatalysis |
Top-N result to add to graph | 6 |
Feasible Synthetic Routes
Disclaimer and Information on In-Vitro Research Products
Please be aware that all articles and product information presented on BenchChem are intended solely for informational purposes. The products available for purchase on BenchChem are specifically designed for in-vitro studies, which are conducted outside of living organisms. In-vitro studies, derived from the Latin term "in glass," involve experiments performed in controlled laboratory settings using cells or tissues. It is important to note that these products are not categorized as medicines or drugs, and they have not received approval from the FDA for the prevention, treatment, or cure of any medical condition, ailment, or disease. We must emphasize that any form of bodily introduction of these products into humans or animals is strictly prohibited by law. It is essential to adhere to these guidelines to ensure compliance with legal and ethical standards in research and experimentation.