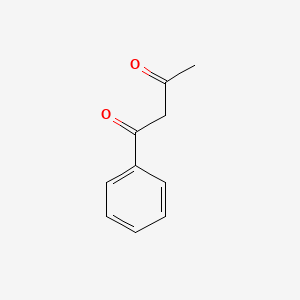
Benzoylacetone
Overview
Description
Mechanism of Action
Target of Action
Benzoylacetone, also known as 1-phenylbutane-1,3-dione, is an organic compound with the nominal formula C6H5C(O)CH2C(O)CH3 . It is a 1,3-dicarbonyl compound and a precursor to many heterocycles, such as pyrazoles . The primary targets of this compound are transition metals and lanthanides, with which its conjugate base (pKa = 8.7) forms stable complexes .
Mode of Action
This compound predominantly exists as the enol tautomer C6H5C(OH)=CHC(O)CH3 . It interacts with its targets, the transition metals and lanthanides, by forming stable complexes . This interaction is facilitated by the conjugate base of this compound, which has a pKa value of 8.7
Biochemical Pathways
As a 1,3-dicarbonyl compound, it is a precursor to many heterocycles, such as pyrazoles . This suggests that it may play a role in the synthesis of these heterocycles and potentially influence related biochemical pathways.
Result of Action
Its ability to form stable complexes with transition metals and lanthanides suggests that it may influence processes involving these elements
Action Environment
The action, efficacy, and stability of this compound can be influenced by various environmental factors. For instance, the pH of the environment can affect the formation of the conjugate base of this compound, which is crucial for its interaction with its targets Additionally, factors such as temperature and the presence of other chemicals can also influence the action of this compound
Biochemical Analysis
Biochemical Properties
Benzoylacetone, as a 1,3-dicarbonyl compound, plays a significant role in biochemical reactions. It is known to form stable complexes with transition metals and lanthanides
Cellular Effects
As a precursor to many heterocycles, it is likely to influence cell function, including impacts on cell signaling pathways, gene expression, and cellular metabolism .
Molecular Mechanism
The molecular mechanism of this compound is complex and multifaceted. It is known to exert its effects at the molecular level through its ability to form stable complexes with transition metals and lanthanides . This suggests potential binding interactions with biomolecules, enzyme inhibition or activation, and changes in gene expression.
Metabolic Pathways
This compound is involved in several metabolic pathways due to its role as a precursor to many heterocycles . The specific enzymes or cofactors it interacts with, and its effects on metabolic flux or metabolite levels, are areas of active research.
Preparation Methods
Synthetic Routes and Reaction Conditions: Benzoylacetone can be synthesized through several methods:
Reaction of Benzene and Diketene: This method involves the reaction of benzene and diketene in the presence of a polymerization inhibitor.
Reaction of Benzoyl Chloride and Ethyl Acetoacetate: In this method, benzoyl chloride reacts with ethyl acetoacetate in the presence of a base to form this compound.
Reaction of Acetophenone and Ethyl Acetate: This involves the reaction of acetophenone with ethyl acetate in the presence of a base.
Industrial Production Methods: The industrial production of this compound typically involves the reaction of benzene and diketene due to its high yield, simplicity in separation and purification processes, and low environmental impact .
Types of Reactions:
Oxidation: this compound can undergo oxidation reactions to form various oxidized products.
Reduction: It can be reduced to form corresponding alcohols or hydrocarbons.
Substitution: this compound can participate in substitution reactions, particularly at the carbonyl groups.
Common Reagents and Conditions:
Oxidation: Common oxidizing agents include potassium permanganate and chromium trioxide.
Reduction: Reduction can be carried out using hydrogen gas in the presence of a catalyst such as Raney nickel.
Substitution: Substitution reactions often involve nucleophiles such as hydrazine or hydroxylamine.
Major Products:
Oxidation: Oxidized derivatives of this compound.
Reduction: Corresponding alcohols or hydrocarbons.
Substitution: Various substituted derivatives depending on the nucleophile used.
Scientific Research Applications
Benzoylacetone has a wide range of applications in scientific research:
Chemistry: It is used as a model compound for studying keto-enol equilibria in aqueous acid and micellar solutions.
Biology: this compound is used in the synthesis of various biologically active compounds.
Medicine: It serves as an intermediate in the synthesis of pharmaceutical compounds.
Industry: this compound is used as a chelating agent and in the production of fragrances and flavors.
Comparison with Similar Compounds
Acetylacetone (2,4-pentanedione): Similar to benzoylacetone, acetylacetone is a 1,3-dicarbonyl compound and exists in an enol form.
Benzylacetone (4-phenyl-2-butanone): This compound is structurally similar but lacks the 1,3-dicarbonyl functionality.
Uniqueness: this compound’s ability to form stable complexes with transition metals and lanthanides sets it apart from other similar compounds . This property makes it particularly valuable in applications requiring strong metal-ligand interactions .
Properties
IUPAC Name |
1-phenylbutane-1,3-dione | |
---|---|---|
Source | PubChem | |
URL | https://pubchem.ncbi.nlm.nih.gov | |
Description | Data deposited in or computed by PubChem | |
InChI |
InChI=1S/C10H10O2/c1-8(11)7-10(12)9-5-3-2-4-6-9/h2-6H,7H2,1H3 | |
Source | PubChem | |
URL | https://pubchem.ncbi.nlm.nih.gov | |
Description | Data deposited in or computed by PubChem | |
InChI Key |
CVBUKMMMRLOKQR-UHFFFAOYSA-N | |
Source | PubChem | |
URL | https://pubchem.ncbi.nlm.nih.gov | |
Description | Data deposited in or computed by PubChem | |
Canonical SMILES |
CC(=O)CC(=O)C1=CC=CC=C1 | |
Source | PubChem | |
URL | https://pubchem.ncbi.nlm.nih.gov | |
Description | Data deposited in or computed by PubChem | |
Molecular Formula |
C10H10O2 | |
Source | PubChem | |
URL | https://pubchem.ncbi.nlm.nih.gov | |
Description | Data deposited in or computed by PubChem | |
DSSTOX Substance ID |
DTXSID3021803 | |
Record name | 1-Benzoylacetone | |
Source | EPA DSSTox | |
URL | https://comptox.epa.gov/dashboard/DTXSID3021803 | |
Description | DSSTox provides a high quality public chemistry resource for supporting improved predictive toxicology. | |
Molecular Weight |
162.18 g/mol | |
Source | PubChem | |
URL | https://pubchem.ncbi.nlm.nih.gov | |
Description | Data deposited in or computed by PubChem | |
Physical Description |
Yellow crystalline powder; [Acros Organics MSDS] | |
Record name | Benzoylacetone | |
Source | Haz-Map, Information on Hazardous Chemicals and Occupational Diseases | |
URL | https://haz-map.com/Agents/20300 | |
Description | Haz-Map® is an occupational health database designed for health and safety professionals and for consumers seeking information about the adverse effects of workplace exposures to chemical and biological agents. | |
Explanation | Copyright (c) 2022 Haz-Map(R). All rights reserved. Unless otherwise indicated, all materials from Haz-Map are copyrighted by Haz-Map(R). No part of these materials, either text or image may be used for any purpose other than for personal use. Therefore, reproduction, modification, storage in a retrieval system or retransmission, in any form or by any means, electronic, mechanical or otherwise, for reasons other than personal use, is strictly prohibited without prior written permission. | |
CAS No. |
93-91-4 | |
Record name | Benzoylacetone | |
Source | CAS Common Chemistry | |
URL | https://commonchemistry.cas.org/detail?cas_rn=93-91-4 | |
Description | CAS Common Chemistry is an open community resource for accessing chemical information. Nearly 500,000 chemical substances from CAS REGISTRY cover areas of community interest, including common and frequently regulated chemicals, and those relevant to high school and undergraduate chemistry classes. This chemical information, curated by our expert scientists, is provided in alignment with our mission as a division of the American Chemical Society. | |
Explanation | The data from CAS Common Chemistry is provided under a CC-BY-NC 4.0 license, unless otherwise stated. | |
Record name | Benzoylacetone | |
Source | ChemIDplus | |
URL | https://pubchem.ncbi.nlm.nih.gov/substance/?source=chemidplus&sourceid=0000093914 | |
Description | ChemIDplus is a free, web search system that provides access to the structure and nomenclature authority files used for the identification of chemical substances cited in National Library of Medicine (NLM) databases, including the TOXNET system. | |
Record name | Benzoylacetone | |
Source | DTP/NCI | |
URL | https://dtp.cancer.gov/dtpstandard/servlet/dwindex?searchtype=NSC&outputformat=html&searchlist=4015 | |
Description | The NCI Development Therapeutics Program (DTP) provides services and resources to the academic and private-sector research communities worldwide to facilitate the discovery and development of new cancer therapeutic agents. | |
Explanation | Unless otherwise indicated, all text within NCI products is free of copyright and may be reused without our permission. Credit the National Cancer Institute as the source. | |
Record name | 1,3-Butanedione, 1-phenyl- | |
Source | EPA Chemicals under the TSCA | |
URL | https://www.epa.gov/chemicals-under-tsca | |
Description | EPA Chemicals under the Toxic Substances Control Act (TSCA) collection contains information on chemicals and their regulations under TSCA, including non-confidential content from the TSCA Chemical Substance Inventory and Chemical Data Reporting. | |
Record name | 1-Benzoylacetone | |
Source | EPA DSSTox | |
URL | https://comptox.epa.gov/dashboard/DTXSID3021803 | |
Description | DSSTox provides a high quality public chemistry resource for supporting improved predictive toxicology. | |
Record name | 1-phenylbutane-1,3-dione | |
Source | European Chemicals Agency (ECHA) | |
URL | https://echa.europa.eu/substance-information/-/substanceinfo/100.002.080 | |
Description | The European Chemicals Agency (ECHA) is an agency of the European Union which is the driving force among regulatory authorities in implementing the EU's groundbreaking chemicals legislation for the benefit of human health and the environment as well as for innovation and competitiveness. | |
Explanation | Use of the information, documents and data from the ECHA website is subject to the terms and conditions of this Legal Notice, and subject to other binding limitations provided for under applicable law, the information, documents and data made available on the ECHA website may be reproduced, distributed and/or used, totally or in part, for non-commercial purposes provided that ECHA is acknowledged as the source: "Source: European Chemicals Agency, http://echa.europa.eu/". Such acknowledgement must be included in each copy of the material. ECHA permits and encourages organisations and individuals to create links to the ECHA website under the following cumulative conditions: Links can only be made to webpages that provide a link to the Legal Notice page. | |
Record name | 1-PHENYL-1,3-BUTANEDIONE | |
Source | FDA Global Substance Registration System (GSRS) | |
URL | https://gsrs.ncats.nih.gov/ginas/app/beta/substances/I3RUV8U115 | |
Description | The FDA Global Substance Registration System (GSRS) enables the efficient and accurate exchange of information on what substances are in regulated products. Instead of relying on names, which vary across regulatory domains, countries, and regions, the GSRS knowledge base makes it possible for substances to be defined by standardized, scientific descriptions. | |
Explanation | Unless otherwise noted, the contents of the FDA website (www.fda.gov), both text and graphics, are not copyrighted. They are in the public domain and may be republished, reprinted and otherwise used freely by anyone without the need to obtain permission from FDA. Credit to the U.S. Food and Drug Administration as the source is appreciated but not required. | |
Synthesis routes and methods I
Procedure details
Synthesis routes and methods II
Procedure details
Retrosynthesis Analysis
AI-Powered Synthesis Planning: Our tool employs the Template_relevance Pistachio, Template_relevance Bkms_metabolic, Template_relevance Pistachio_ringbreaker, Template_relevance Reaxys, Template_relevance Reaxys_biocatalysis model, leveraging a vast database of chemical reactions to predict feasible synthetic routes.
One-Step Synthesis Focus: Specifically designed for one-step synthesis, it provides concise and direct routes for your target compounds, streamlining the synthesis process.
Accurate Predictions: Utilizing the extensive PISTACHIO, BKMS_METABOLIC, PISTACHIO_RINGBREAKER, REAXYS, REAXYS_BIOCATALYSIS database, our tool offers high-accuracy predictions, reflecting the latest in chemical research and data.
Strategy Settings
Precursor scoring | Relevance Heuristic |
---|---|
Min. plausibility | 0.01 |
Model | Template_relevance |
Template Set | Pistachio/Bkms_metabolic/Pistachio_ringbreaker/Reaxys/Reaxys_biocatalysis |
Top-N result to add to graph | 6 |
Feasible Synthetic Routes
Disclaimer and Information on In-Vitro Research Products
Please be aware that all articles and product information presented on BenchChem are intended solely for informational purposes. The products available for purchase on BenchChem are specifically designed for in-vitro studies, which are conducted outside of living organisms. In-vitro studies, derived from the Latin term "in glass," involve experiments performed in controlled laboratory settings using cells or tissues. It is important to note that these products are not categorized as medicines or drugs, and they have not received approval from the FDA for the prevention, treatment, or cure of any medical condition, ailment, or disease. We must emphasize that any form of bodily introduction of these products into humans or animals is strictly prohibited by law. It is essential to adhere to these guidelines to ensure compliance with legal and ethical standards in research and experimentation.