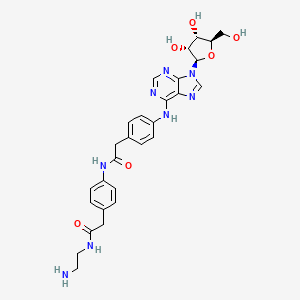
Adenosine amine congener
Overview
Description
Adenosine amine congener is a synthetic compound known for its selective agonistic activity on the A1 adenosine receptor. This compound has garnered significant attention due to its potential therapeutic applications, particularly in neuroprotection and otoprotection. This compound has been shown to ameliorate noise- and cisplatin-induced cochlear injury, making it a promising candidate for treating hearing loss and other neurological conditions .
Mechanism of Action
Target of Action
Adenosine amine congener (ADAC) is a selective agonist for the A1 adenosine receptor . This receptor is one of the four G protein-coupled receptors for adenosine and is widely distributed in the body . It plays a modulatory role due to its effects in many organs and tissues .
Mode of Action
ADAC, as an A1 adenosine receptor agonist, interacts with its targets by binding to the A1 adenosine receptors . This interaction leads to a decrease in conduction time in the atrioventricular node of the heart . The conduction time is decreased by inducing potassium efflux and inhibiting calcium influx through channels in nerve cells, leading to hyperpolarization and an increased threshold for calcium-dependent action .
Biochemical Pathways
The A1 adenosine receptor, when activated by ADAC, modulates numerous normal and pathological processes through signaling pathways downstream from its coupled Gi protein . These effects include bradycardia, inhibition of neurotransmitter release, inhibition of lipolysis in adipocytes, inhibition of renal excretion, and induction of smooth muscle contraction .
Pharmacokinetics
ADAC is detected in cochlear perilymph within two minutes following intravenous administration and remains in perilymph above its minimal effective concentration for at least two hours . Pharmacokinetic studies have demonstrated a short (5 minutes) half-life of ADAC in plasma after intravenous administration without detection of degradation products .
Result of Action
ADAC has been shown to ameliorate noise- and cisplatin-induced cochlear injury . It has been demonstrated that ADAC mitigates noise-induced hearing loss in a dose- and time-dependent manner . ADAC also has neuroprotective effects .
Action Environment
The pharmacokinetic pattern of ADAC is significantly altered by exposure to noise, suggesting transient changes in the permeability of the blood-labyrinth barrier and/or cochlear blood flow . This indicates that environmental factors such as noise can influence the action, efficacy, and stability of ADAC .
Biochemical Analysis
Biochemical Properties
ADAC interacts with the A1 adenosine receptor, a G protein-coupled receptor, which is widely distributed in the body . This interaction triggers various biochemical reactions. For instance, ADAC has been shown to ameliorate noise- and cisplatin-induced cochlear injury, indicating its role in biochemical reactions related to cellular protection .
Cellular Effects
ADAC has a profound impact on various types of cells and cellular processes. It influences cell function by impacting cell signaling pathways, gene expression, and cellular metabolism . For example, in the case of noise-induced cochlear injury, ADAC has been shown to have a dose-dependent rescue effect, improving hearing thresholds in rats .
Molecular Mechanism
ADAC exerts its effects at the molecular level primarily through its interaction with the A1 adenosine receptor . This interaction can lead to various outcomes, including the inhibition or activation of enzymes and changes in gene expression .
Temporal Effects in Laboratory Settings
The effects of ADAC change over time in laboratory settings. Studies have shown that ADAC is most effective in the first 24 hours after noise exposure at doses greater than 50 μg/kg, providing significant protection . Furthermore, ADAC has a short half-life of 5 minutes in plasma after intravenous administration, indicating its rapid metabolism .
Dosage Effects in Animal Models
The effects of ADAC vary with different dosages in animal models. For instance, in a study on noise-induced cochlear injury in rats, ADAC was administered at doses ranging from 25 to 300 μg/kg. The study found that ADAC was most effective at doses greater than 50 μg/kg .
Metabolic Pathways
ADAC is involved in the adenosine metabolic pathway. Adenosine is formed due to intracellular ATP breakdown into ADP . ADAC, being an adenosine receptor agonist, plays a role in this metabolic pathway.
Transport and Distribution
The transport and distribution of ADAC within cells and tissues are likely facilitated by specific transporters or binding proteins, similar to other adenosine receptor agonists
Subcellular Localization
The subcellular localization of ADAC is likely to be influenced by its interaction with the A1 adenosine receptor, which is a membrane-bound receptor
Preparation Methods
Synthetic Routes and Reaction Conditions: The synthesis of adenosine amine congener typically involves the modification of adenosine through a series of chemical reactions. One common method includes the alkylation of adenosine with an appropriate amine under controlled conditions. The reaction is usually carried out in an organic solvent such as dimethyl sulfoxide (DMSO) or acetonitrile, with a base like potassium carbonate to facilitate the reaction. The product is then purified using chromatographic techniques to achieve high purity.
Industrial Production Methods: Industrial production of this compound follows similar synthetic routes but on a larger scale. The process involves optimizing reaction conditions to maximize yield and purity while minimizing costs and environmental impact. Advanced purification techniques, such as high-performance liquid chromatography (HPLC), are employed to ensure the compound meets stringent quality standards required for research and potential therapeutic use.
Chemical Reactions Analysis
Types of Reactions: Adenosine amine congener undergoes various chemical reactions, including:
Oxidation: This reaction can modify the functional groups on the molecule, potentially altering its biological activity.
Reduction: Reduction reactions can be used to modify the compound’s structure, affecting its receptor binding affinity.
Common Reagents and Conditions:
Oxidation: Reagents such as hydrogen peroxide or potassium permanganate under acidic or basic conditions.
Reduction: Common reducing agents include sodium borohydride or lithium aluminum hydride.
Substitution: Nucleophiles like amines or thiols in the presence of a suitable leaving group and a base.
Major Products: The major products formed from these reactions depend on the specific reagents and conditions used. For example, oxidation may yield hydroxylated derivatives, while substitution reactions can produce a variety of functionalized analogs.
Scientific Research Applications
Adenosine amine congener has a wide range of applications in scientific research:
Chemistry: Used as a tool to study adenosine receptor interactions and signaling pathways.
Biology: Investigated for its role in cellular processes such as apoptosis, oxidative stress, and neurotransmitter release.
Medicine: Explored for its neuroprotective and otoprotective properties, particularly in conditions like hearing loss and neurodegenerative diseases.
Industry: Potential applications in the development of therapeutic agents targeting adenosine receptors.
Comparison with Similar Compounds
Adenosine amine congener is unique in its high selectivity for A1 adenosine receptors compared to other adenosine receptor agonists. Similar compounds include:
N6-Cyclopentyladenosine (CPA): Another selective A1 receptor agonist but with different pharmacokinetic properties.
N6-Cyclohexyladenosine (CHA): Known for its potent A1 receptor activity but less selective than this compound.
2-Chloroadenosine: A non-selective adenosine receptor agonist with broader biological effects.
This compound’s specificity and efficacy make it a valuable compound for research and potential therapeutic applications.
Properties
IUPAC Name |
N-(2-aminoethyl)-2-[4-[[2-[4-[[9-[(2R,3R,4S,5R)-3,4-dihydroxy-5-(hydroxymethyl)oxolan-2-yl]purin-6-yl]amino]phenyl]acetyl]amino]phenyl]acetamide | |
---|---|---|
Source | PubChem | |
URL | https://pubchem.ncbi.nlm.nih.gov | |
Description | Data deposited in or computed by PubChem | |
InChI |
InChI=1S/C28H32N8O6/c29-9-10-30-21(38)11-16-1-5-18(6-2-16)34-22(39)12-17-3-7-19(8-4-17)35-26-23-27(32-14-31-26)36(15-33-23)28-25(41)24(40)20(13-37)42-28/h1-8,14-15,20,24-25,28,37,40-41H,9-13,29H2,(H,30,38)(H,34,39)(H,31,32,35)/t20-,24-,25-,28-/m1/s1 | |
Source | PubChem | |
URL | https://pubchem.ncbi.nlm.nih.gov | |
Description | Data deposited in or computed by PubChem | |
InChI Key |
JFRJCQJVFMHZOO-QZHHGCDDSA-N | |
Source | PubChem | |
URL | https://pubchem.ncbi.nlm.nih.gov | |
Description | Data deposited in or computed by PubChem | |
Canonical SMILES |
C1=CC(=CC=C1CC(=O)NC2=CC=C(C=C2)CC(=O)NCCN)NC3=C4C(=NC=N3)N(C=N4)C5C(C(C(O5)CO)O)O | |
Source | PubChem | |
URL | https://pubchem.ncbi.nlm.nih.gov | |
Description | Data deposited in or computed by PubChem | |
Isomeric SMILES |
C1=CC(=CC=C1CC(=O)NC2=CC=C(C=C2)CC(=O)NCCN)NC3=C4C(=NC=N3)N(C=N4)[C@H]5[C@@H]([C@@H]([C@H](O5)CO)O)O | |
Source | PubChem | |
URL | https://pubchem.ncbi.nlm.nih.gov | |
Description | Data deposited in or computed by PubChem | |
Molecular Formula |
C28H32N8O6 | |
Source | PubChem | |
URL | https://pubchem.ncbi.nlm.nih.gov | |
Description | Data deposited in or computed by PubChem | |
DSSTOX Substance ID |
DTXSID60914331 | |
Record name | N-{4-[2-(4-{2-[(2-Aminoethyl)imino]-2-hydroxyethyl}anilino)-2-oxoethyl]phenyl}-9-pentofuranosyl-9H-purin-6-amine | |
Source | EPA DSSTox | |
URL | https://comptox.epa.gov/dashboard/DTXSID60914331 | |
Description | DSSTox provides a high quality public chemistry resource for supporting improved predictive toxicology. | |
Molecular Weight |
576.6 g/mol | |
Source | PubChem | |
URL | https://pubchem.ncbi.nlm.nih.gov | |
Description | Data deposited in or computed by PubChem | |
CAS No. |
96760-69-9 | |
Record name | Adenosine amine congener | |
Source | ChemIDplus | |
URL | https://pubchem.ncbi.nlm.nih.gov/substance/?source=chemidplus&sourceid=0096760699 | |
Description | ChemIDplus is a free, web search system that provides access to the structure and nomenclature authority files used for the identification of chemical substances cited in National Library of Medicine (NLM) databases, including the TOXNET system. | |
Record name | N-{4-[2-(4-{2-[(2-Aminoethyl)imino]-2-hydroxyethyl}anilino)-2-oxoethyl]phenyl}-9-pentofuranosyl-9H-purin-6-amine | |
Source | EPA DSSTox | |
URL | https://comptox.epa.gov/dashboard/DTXSID60914331 | |
Description | DSSTox provides a high quality public chemistry resource for supporting improved predictive toxicology. | |
Retrosynthesis Analysis
AI-Powered Synthesis Planning: Our tool employs the Template_relevance Pistachio, Template_relevance Bkms_metabolic, Template_relevance Pistachio_ringbreaker, Template_relevance Reaxys, Template_relevance Reaxys_biocatalysis model, leveraging a vast database of chemical reactions to predict feasible synthetic routes.
One-Step Synthesis Focus: Specifically designed for one-step synthesis, it provides concise and direct routes for your target compounds, streamlining the synthesis process.
Accurate Predictions: Utilizing the extensive PISTACHIO, BKMS_METABOLIC, PISTACHIO_RINGBREAKER, REAXYS, REAXYS_BIOCATALYSIS database, our tool offers high-accuracy predictions, reflecting the latest in chemical research and data.
Strategy Settings
Precursor scoring | Relevance Heuristic |
---|---|
Min. plausibility | 0.01 |
Model | Template_relevance |
Template Set | Pistachio/Bkms_metabolic/Pistachio_ringbreaker/Reaxys/Reaxys_biocatalysis |
Top-N result to add to graph | 6 |
Feasible Synthetic Routes
Disclaimer and Information on In-Vitro Research Products
Please be aware that all articles and product information presented on BenchChem are intended solely for informational purposes. The products available for purchase on BenchChem are specifically designed for in-vitro studies, which are conducted outside of living organisms. In-vitro studies, derived from the Latin term "in glass," involve experiments performed in controlled laboratory settings using cells or tissues. It is important to note that these products are not categorized as medicines or drugs, and they have not received approval from the FDA for the prevention, treatment, or cure of any medical condition, ailment, or disease. We must emphasize that any form of bodily introduction of these products into humans or animals is strictly prohibited by law. It is essential to adhere to these guidelines to ensure compliance with legal and ethical standards in research and experimentation.