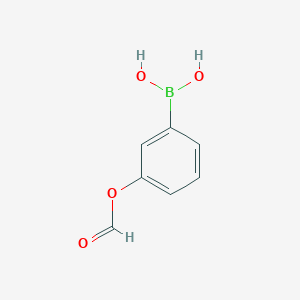
3-Carboxyphenylboronic acid
Overview
Description
Mechanism of Action
Target of Action
3-Carboxyphenylboronic acid is a biochemical reagent . It primarily targets the respiratory system
Mode of Action
It is known to be used as a substrate in the preparation of various compounds . For instance, it can react with bromoaniline through the Suzuki-Miyaura coupling reaction to produce biaryl derivatives .
Biochemical Pathways
Its role as a substrate in the suzuki-miyaura coupling reaction suggests that it may influence pathways involving biaryl derivatives .
Result of Action
Its use as a substrate in the preparation of biaryl derivatives suggests that it may play a role in the synthesis of these compounds .
Action Environment
The action of this compound can be influenced by environmental factors. For instance, it should be stored in a dry, cool, and well-ventilated place . This suggests that moisture, temperature, and ventilation could potentially affect its stability and efficacy.
Biochemical Analysis
Biochemical Properties
3-Carboxyphenylboronic acid plays a significant role in biochemical reactions, particularly in the formation of biaryl derivatives through the Suzuki-Miyaura coupling reaction . This reaction involves the interaction of this compound with bromoaniline, leading to the formation of biaryl compounds. The compound also interacts with enzymes and proteins that facilitate the coupling reaction, such as palladium catalysts. These interactions are crucial for the successful formation of the desired biaryl products.
Cellular Effects
This compound influences various cellular processes, including cell signaling pathways, gene expression, and cellular metabolism. The compound has been shown to affect the activity of certain enzymes involved in these processes, leading to changes in cell function. For example, this compound can modulate the activity of enzymes involved in the synthesis of biaryl derivatives, thereby impacting cellular metabolism and gene expression . Additionally, the compound’s ability to interact with proteins and enzymes can influence cell signaling pathways, leading to alterations in cellular responses.
Molecular Mechanism
The molecular mechanism of this compound involves its interaction with biomolecules, such as enzymes and proteins. The compound can bind to specific sites on these biomolecules, leading to enzyme inhibition or activation. For instance, this compound can inhibit the activity of certain enzymes involved in the synthesis of biaryl derivatives, thereby affecting the overall reaction . Additionally, the compound can induce changes in gene expression by interacting with transcription factors and other regulatory proteins.
Temporal Effects in Laboratory Settings
In laboratory settings, the effects of this compound can change over time due to factors such as stability and degradation. The compound is relatively stable under standard laboratory conditions, but it can degrade over time, leading to a decrease in its effectiveness . Long-term studies have shown that this compound can have lasting effects on cellular function, particularly in in vitro and in vivo experiments. These effects are often dependent on the concentration and duration of exposure to the compound.
Dosage Effects in Animal Models
The effects of this compound vary with different dosages in animal models. At low doses, the compound can effectively modulate enzyme activity and cellular processes without causing significant adverse effects . At high doses, this compound can exhibit toxic effects, including enzyme inhibition and disruption of cellular function. These threshold effects are important considerations in the design of experiments and the interpretation of results.
Metabolic Pathways
This compound is involved in various metabolic pathways, particularly those related to the synthesis of biaryl derivatives. The compound interacts with enzymes and cofactors that facilitate these reactions, leading to changes in metabolic flux and metabolite levels . For example, this compound can influence the activity of enzymes involved in the Suzuki-Miyaura coupling reaction, thereby affecting the overall metabolic pathway.
Transport and Distribution
Within cells and tissues, this compound is transported and distributed through interactions with transporters and binding proteins. These interactions can influence the localization and accumulation of the compound, affecting its overall activity and function . For instance, this compound can bind to specific transporters that facilitate its movement across cell membranes, leading to its distribution within various cellular compartments.
Subcellular Localization
The subcellular localization of this compound is influenced by targeting signals and post-translational modifications that direct it to specific compartments or organelles. These localization signals are crucial for the compound’s activity and function, as they determine its interactions with specific biomolecules . For example, this compound can be directed to the nucleus, where it interacts with transcription factors and other regulatory proteins, influencing gene expression and cellular responses.
Preparation Methods
3-boronobenzoic acid can be synthesized through several methods. One common synthetic route involves the reaction of benzoic acid with boron-containing reagents under specific conditions. For instance, the Suzuki-Miyaura coupling reaction is often employed, where benzoic acid derivatives react with boronic acids or boronate esters in the presence of a palladium catalyst and a base. The reaction typically occurs in an organic solvent such as ethanol or tetrahydrofuran at elevated temperatures .
Chemical Reactions Analysis
3-boronobenzoic acid undergoes various chemical reactions, including:
Oxidation: This compound can be oxidized to form different products depending on the oxidizing agent and conditions used.
Reduction: Reduction reactions can convert 3-boronobenzoic acid into other boron-containing compounds.
Substitution: The boronic acid group can participate in substitution reactions, where it is replaced by other functional groups.
Coupling Reactions: The Suzuki-Miyaura coupling reaction is a notable example, where 3-boronobenzoic acid reacts with halogenated compounds to form biaryl derivatives.
Common reagents used in these reactions include palladium catalysts, bases like potassium carbonate, and solvents such as ethanol and tetrahydrofuran. The major products formed from these reactions vary based on the specific reaction conditions and reagents used.
Scientific Research Applications
3-boronobenzoic acid has a wide range of applications in scientific research:
Biology: The compound is utilized in the development of boron-containing drugs and as a tool in biochemical assays.
Medicine: Research is ongoing into its potential use in boron neutron capture therapy (BNCT) for cancer treatment.
Comparison with Similar Compounds
3-boronobenzoic acid can be compared to other boronic acids, such as:
Phenylboronic acid: Similar in structure but lacks the carboxyl group, making it less versatile in certain reactions.
4-boronobenzoic acid: Another positional isomer with the boronic acid group at a different location on the benzene ring, leading to different reactivity and applications.
2-boronobenzoic acid: Similar to 3-boronobenzoic acid but with the boronic acid group in the ortho position, affecting its chemical behavior and uses.
The uniqueness of 3-boronobenzoic acid lies in its specific substitution pattern, which imparts distinct reactivity and makes it suitable for a variety of specialized applications.
Properties
IUPAC Name |
3-boronobenzoic acid | |
---|---|---|
Source | PubChem | |
URL | https://pubchem.ncbi.nlm.nih.gov | |
Description | Data deposited in or computed by PubChem | |
InChI |
InChI=1S/C7H7BO4/c9-7(10)5-2-1-3-6(4-5)8(11)12/h1-4,11-12H,(H,9,10) | |
Source | PubChem | |
URL | https://pubchem.ncbi.nlm.nih.gov | |
Description | Data deposited in or computed by PubChem | |
InChI Key |
DBVFWZMQJQMJCB-UHFFFAOYSA-N | |
Source | PubChem | |
URL | https://pubchem.ncbi.nlm.nih.gov | |
Description | Data deposited in or computed by PubChem | |
Canonical SMILES |
B(C1=CC(=CC=C1)C(=O)O)(O)O | |
Source | PubChem | |
URL | https://pubchem.ncbi.nlm.nih.gov | |
Description | Data deposited in or computed by PubChem | |
Molecular Formula |
C7H7BO4 | |
Source | PubChem | |
URL | https://pubchem.ncbi.nlm.nih.gov | |
Description | Data deposited in or computed by PubChem | |
Molecular Weight |
165.94 g/mol | |
Source | PubChem | |
URL | https://pubchem.ncbi.nlm.nih.gov | |
Description | Data deposited in or computed by PubChem | |
CAS No. |
25487-66-5 | |
Record name | 3-Carboxyphenylboronic acid | |
Source | CAS Common Chemistry | |
URL | https://commonchemistry.cas.org/detail?cas_rn=25487-66-5 | |
Description | CAS Common Chemistry is an open community resource for accessing chemical information. Nearly 500,000 chemical substances from CAS REGISTRY cover areas of community interest, including common and frequently regulated chemicals, and those relevant to high school and undergraduate chemistry classes. This chemical information, curated by our expert scientists, is provided in alignment with our mission as a division of the American Chemical Society. | |
Explanation | The data from CAS Common Chemistry is provided under a CC-BY-NC 4.0 license, unless otherwise stated. | |
Retrosynthesis Analysis
AI-Powered Synthesis Planning: Our tool employs the Template_relevance Pistachio, Template_relevance Bkms_metabolic, Template_relevance Pistachio_ringbreaker, Template_relevance Reaxys, Template_relevance Reaxys_biocatalysis model, leveraging a vast database of chemical reactions to predict feasible synthetic routes.
One-Step Synthesis Focus: Specifically designed for one-step synthesis, it provides concise and direct routes for your target compounds, streamlining the synthesis process.
Accurate Predictions: Utilizing the extensive PISTACHIO, BKMS_METABOLIC, PISTACHIO_RINGBREAKER, REAXYS, REAXYS_BIOCATALYSIS database, our tool offers high-accuracy predictions, reflecting the latest in chemical research and data.
Strategy Settings
Precursor scoring | Relevance Heuristic |
---|---|
Min. plausibility | 0.01 |
Model | Template_relevance |
Template Set | Pistachio/Bkms_metabolic/Pistachio_ringbreaker/Reaxys/Reaxys_biocatalysis |
Top-N result to add to graph | 6 |
Feasible Synthetic Routes
Disclaimer and Information on In-Vitro Research Products
Please be aware that all articles and product information presented on BenchChem are intended solely for informational purposes. The products available for purchase on BenchChem are specifically designed for in-vitro studies, which are conducted outside of living organisms. In-vitro studies, derived from the Latin term "in glass," involve experiments performed in controlled laboratory settings using cells or tissues. It is important to note that these products are not categorized as medicines or drugs, and they have not received approval from the FDA for the prevention, treatment, or cure of any medical condition, ailment, or disease. We must emphasize that any form of bodily introduction of these products into humans or animals is strictly prohibited by law. It is essential to adhere to these guidelines to ensure compliance with legal and ethical standards in research and experimentation.