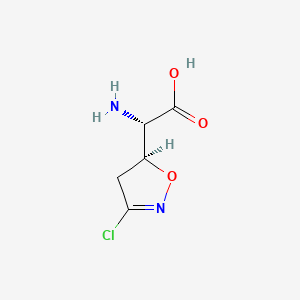
Acivicin
Overview
Description
Acivicin is a natural product produced by the bacterium Streptomyces sviceus. It is known for its diverse biological activities, including anti-cancer and anti-parasitic properties . This compound was discovered in 1972 and has been extensively studied for its potential application as an anti-tumor drug . its clinical application was limited due to unacceptable toxicity .
Mechanism of Action
Target of Action
Acivicin, a natural product produced by Streptomyces sviceus, primarily targets aldehyde dehydrogenases , specifically ALDH4A1 . Aldehyde dehydrogenases are crucial enzymes involved in cellular detoxification, differentiation, and drug resistance .
Mode of Action
This compound inhibits the activity of ALDH4A1 by binding to its catalytic site . This interaction disrupts the normal function of ALDH4A1, leading to a significant impact on cellular processes .
Biochemical Pathways
This compound is an analog of glutamine and interferes with glutamate metabolism . It inhibits several glutamine-dependent amidotransferases, including CTP synthase, carbamoyl phosphate synthetase II, and XMP aminase . These enzymes are involved in purine and pyrimidine metabolism, and their inhibition by this compound leads to decreases in cellular CTP and GTP levels .
Result of Action
The inhibition of ALDH4A1 by this compound results in a severe inhibition of cell growth . This might provide an explanation for the cytotoxic effects of this compound . The clinical application of this compound in cancer treatment was explored but failed due to unacceptable toxicity .
Biochemical Analysis
Biochemical Properties
Acivicin is known to interfere with glutamate metabolism and inhibit glutamate-dependent synthesis of enzymes . It inhibits several glutamine-dependent amidotransferases, including CTP synthase, carbamoyl phosphate synthetase II, and XMP aminase, which are involved in purine and pyrimidine metabolism .
Cellular Effects
This compound has been shown to have significant effects on various types of cells. In vitro studies confirmed the inactivation of several glutamine-dependent amidotransferases, leading to corresponding decreases in cellular CTP and GTP levels . This interference with glutamate metabolism and inhibition of enzyme synthesis potentially makes this compound helpful in the treatment of solid tumors .
Molecular Mechanism
This compound exerts its effects at the molecular level through several mechanisms. It reacts according to an addition–elimination mechanism with nucleophilic serine or cysteine active sites by displacement of the chlorine atom . It also inhibits specific aldehyde dehydrogenases, particularly ALDH4A1, by binding to the catalytic site .
Metabolic Pathways
This compound is involved in several metabolic pathways. It inhibits several glutamine-dependent amidotransferases, which are involved in purine and pyrimidine metabolism . It also inhibits gamma-glutamyltranspeptidase, an enzyme that plays an important role in glutathione metabolism and in amino acid transport by cells .
Transport and Distribution
It’s suggested that the uptake of this compound might be mediated by the L-amino acid transport system .
Preparation Methods
Acivicin is synthesized through a fermentation process involving Streptomyces sviceus . The core structure of this compound includes a 4-chloroisoxazole motif, which is electrophilic and reacts with nucleophilic serine or cysteine active sites by displacing the chlorine atom . Industrial production methods involve optimizing the fermentation conditions to maximize yield and purity .
Chemical Reactions Analysis
Acivicin undergoes several types of chemical reactions, including:
Substitution Reactions: The chlorine atom in the 4-chloroisoxazole motif can be displaced by nucleophiles such as serine or cysteine.
Inhibition Reactions: This compound inhibits several glutamine-dependent amidotransferases, including CTP synthase, carbamoyl phosphate synthetase II, and XMP aminase.
Common reagents and conditions used in these reactions include nucleophiles like serine and cysteine, and the reactions typically occur under physiological conditions . The major products formed from these reactions are the inhibited enzymes and the corresponding displaced chlorine atom .
Scientific Research Applications
Acivicin has been extensively studied for its scientific research applications, including:
Chemistry: This compound is used as a tool compound to study enzyme inhibition and protein interactions.
Biology: It is used to investigate the role of glutamine-dependent amidotransferases in cellular metabolism.
Medicine: This compound has been evaluated for cancer therapy and has shown potential in inhibiting the growth of human pancreatic carcinoma cells.
Comparison with Similar Compounds
Acivicin is unique due to its 4-chloroisoxazole motif and its ability to inhibit multiple glutamine-dependent amidotransferases . Similar compounds include:
Ibotenic Acid: A neurotoxic agent with a structural relationship to this compound.
Muscimol: Another neurotoxic agent related to this compound.
4-Bromo-Isoxazole Probes: These compounds have a similar core structure to this compound and show a preference for reacting with the active site cysteine of aldehyde dehydrogenases.
This compound’s uniqueness lies in its broad spectrum of biological activities and its ability to inhibit multiple enzymes involved in purine and pyrimidine metabolism .
Properties
IUPAC Name |
(2S)-2-amino-2-[(5S)-3-chloro-4,5-dihydro-1,2-oxazol-5-yl]acetic acid | |
---|---|---|
Source | PubChem | |
URL | https://pubchem.ncbi.nlm.nih.gov | |
Description | Data deposited in or computed by PubChem | |
InChI |
InChI=1S/C5H7ClN2O3/c6-3-1-2(11-8-3)4(7)5(9)10/h2,4H,1,7H2,(H,9,10)/t2-,4-/m0/s1 | |
Source | PubChem | |
URL | https://pubchem.ncbi.nlm.nih.gov | |
Description | Data deposited in or computed by PubChem | |
InChI Key |
QAWIHIJWNYOLBE-OKKQSCSOSA-N | |
Source | PubChem | |
URL | https://pubchem.ncbi.nlm.nih.gov | |
Description | Data deposited in or computed by PubChem | |
Canonical SMILES |
C1C(ON=C1Cl)C(C(=O)O)N | |
Source | PubChem | |
URL | https://pubchem.ncbi.nlm.nih.gov | |
Description | Data deposited in or computed by PubChem | |
Isomeric SMILES |
C1[C@H](ON=C1Cl)[C@@H](C(=O)O)N | |
Source | PubChem | |
URL | https://pubchem.ncbi.nlm.nih.gov | |
Description | Data deposited in or computed by PubChem | |
Molecular Formula |
C5H7ClN2O3 | |
Source | PubChem | |
URL | https://pubchem.ncbi.nlm.nih.gov | |
Description | Data deposited in or computed by PubChem | |
DSSTOX Substance ID |
DTXSID0046010 | |
Record name | Acivicin | |
Source | EPA DSSTox | |
URL | https://comptox.epa.gov/dashboard/DTXSID0046010 | |
Description | DSSTox provides a high quality public chemistry resource for supporting improved predictive toxicology. | |
Molecular Weight |
178.57 g/mol | |
Source | PubChem | |
URL | https://pubchem.ncbi.nlm.nih.gov | |
Description | Data deposited in or computed by PubChem | |
Solubility |
Water 17.9 - 18.2 (mg/mL), 0.1 M Citrate buffer pH 4.3 17.1 - 25.7 (mg/mL), 0.1 M Borate buffer pH 9.0 8.7 - 13.0 (mg/mL), 0.1 N HCl 31 - 35 (mg/mL), 0.1 N NaOH 34.4 - 36.8 (mg/mL), 95% Ethanol 0.8 (mg/mL), 10% Ethanol 8.2 - 16.4 (mg/mL), Methanol 0.8 (mg/mL), Chloroform 0.9 (mg/mL) | |
Record name | ACIVICIN | |
Source | NCI Investigational Drugs | |
URL | http://dtp.nci.nih.gov/NCI-InvestigationalDrugsCI92/163501%20(1992).txt | |
Description | An investigational drug is one that is under study but does not have permission from the U.S. Food and Drug Administration (FDA) to be legally marketed and sold in the United States. NCI provides the investigational drug to the physicians who are participating in clinical trials or TRC protocols. For more information please visit NCI investigational drug website: https://www.cancer.gov/about-cancer/treatment/drugs/investigational-drug-access-fact-sheet | |
CAS No. |
42228-92-2 | |
Record name | Acivicin | |
Source | CAS Common Chemistry | |
URL | https://commonchemistry.cas.org/detail?cas_rn=42228-92-2 | |
Description | CAS Common Chemistry is an open community resource for accessing chemical information. Nearly 500,000 chemical substances from CAS REGISTRY cover areas of community interest, including common and frequently regulated chemicals, and those relevant to high school and undergraduate chemistry classes. This chemical information, curated by our expert scientists, is provided in alignment with our mission as a division of the American Chemical Society. | |
Explanation | The data from CAS Common Chemistry is provided under a CC-BY-NC 4.0 license, unless otherwise stated. | |
Record name | Acivicin [USAN:INN] | |
Source | ChemIDplus | |
URL | https://pubchem.ncbi.nlm.nih.gov/substance/?source=chemidplus&sourceid=0042228922 | |
Description | ChemIDplus is a free, web search system that provides access to the structure and nomenclature authority files used for the identification of chemical substances cited in National Library of Medicine (NLM) databases, including the TOXNET system. | |
Record name | acivicin | |
Source | DTP/NCI | |
URL | https://dtp.cancer.gov/dtpstandard/servlet/dwindex?searchtype=NSC&outputformat=html&searchlist=163501 | |
Description | The NCI Development Therapeutics Program (DTP) provides services and resources to the academic and private-sector research communities worldwide to facilitate the discovery and development of new cancer therapeutic agents. | |
Explanation | Unless otherwise indicated, all text within NCI products is free of copyright and may be reused without our permission. Credit the National Cancer Institute as the source. | |
Record name | Acivicin | |
Source | EPA DSSTox | |
URL | https://comptox.epa.gov/dashboard/DTXSID0046010 | |
Description | DSSTox provides a high quality public chemistry resource for supporting improved predictive toxicology. | |
Record name | ACIVICIN | |
Source | FDA Global Substance Registration System (GSRS) | |
URL | https://gsrs.ncats.nih.gov/ginas/app/beta/substances/O0X60K76I6 | |
Description | The FDA Global Substance Registration System (GSRS) enables the efficient and accurate exchange of information on what substances are in regulated products. Instead of relying on names, which vary across regulatory domains, countries, and regions, the GSRS knowledge base makes it possible for substances to be defined by standardized, scientific descriptions. | |
Explanation | Unless otherwise noted, the contents of the FDA website (www.fda.gov), both text and graphics, are not copyrighted. They are in the public domain and may be republished, reprinted and otherwise used freely by anyone without the need to obtain permission from FDA. Credit to the U.S. Food and Drug Administration as the source is appreciated but not required. | |
Retrosynthesis Analysis
AI-Powered Synthesis Planning: Our tool employs the Template_relevance Pistachio, Template_relevance Bkms_metabolic, Template_relevance Pistachio_ringbreaker, Template_relevance Reaxys, Template_relevance Reaxys_biocatalysis model, leveraging a vast database of chemical reactions to predict feasible synthetic routes.
One-Step Synthesis Focus: Specifically designed for one-step synthesis, it provides concise and direct routes for your target compounds, streamlining the synthesis process.
Accurate Predictions: Utilizing the extensive PISTACHIO, BKMS_METABOLIC, PISTACHIO_RINGBREAKER, REAXYS, REAXYS_BIOCATALYSIS database, our tool offers high-accuracy predictions, reflecting the latest in chemical research and data.
Strategy Settings
Precursor scoring | Relevance Heuristic |
---|---|
Min. plausibility | 0.01 |
Model | Template_relevance |
Template Set | Pistachio/Bkms_metabolic/Pistachio_ringbreaker/Reaxys/Reaxys_biocatalysis |
Top-N result to add to graph | 6 |
Feasible Synthetic Routes
Disclaimer and Information on In-Vitro Research Products
Please be aware that all articles and product information presented on BenchChem are intended solely for informational purposes. The products available for purchase on BenchChem are specifically designed for in-vitro studies, which are conducted outside of living organisms. In-vitro studies, derived from the Latin term "in glass," involve experiments performed in controlled laboratory settings using cells or tissues. It is important to note that these products are not categorized as medicines or drugs, and they have not received approval from the FDA for the prevention, treatment, or cure of any medical condition, ailment, or disease. We must emphasize that any form of bodily introduction of these products into humans or animals is strictly prohibited by law. It is essential to adhere to these guidelines to ensure compliance with legal and ethical standards in research and experimentation.