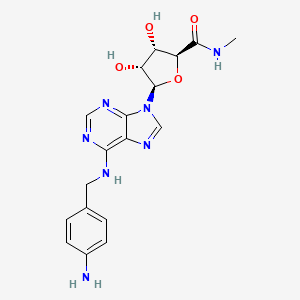
AB-Meca
Overview
Description
Mechanism of Action
Target of Action
AB-Meca is a high-affinity agonist for the A3 adenosine receptor . The A3 adenosine receptor is a G protein-coupled receptor that plays a crucial role in various physiological and pathological processes .
Mode of Action
This compound interacts with the A3 adenosine receptor, enhancing its activity . This interaction results in a change in the receptor’s conformation, which can influence the receptor’s ability to bind to other molecules and transmit signals . The binding of this compound to the A3 adenosine receptor is thought to slow the kinetics of orthosteric ligand binding, reducing the rate of formation of ligand-receptor complexes .
Biochemical Pathways
The activation of the A3 adenosine receptor by this compound can affect various biochemical pathways. For instance, it can enhance plasma histamine levels . Histamine is a key mediator of inflammation and allergic reactions, suggesting that this compound could potentially influence these processes.
Pharmacokinetics
The compound’s high affinity for the a3 adenosine receptor suggests that it may have good bioavailability .
Result of Action
The activation of the A3 adenosine receptor by this compound can have various molecular and cellular effects. For example, it can enhance plasma histamine levels , which can influence immune responses and inflammation. Additionally, this compound has been shown to have anti-proliferative effects on various types of tumors .
Biochemical Analysis
Biochemical Properties
AB-Meca plays a crucial role in biochemical reactions by selectively binding to the adenosine A3 receptor. This receptor is a G protein-coupled receptor that is involved in various physiological processes. This compound interacts with several enzymes, proteins, and other biomolecules, including adenylate cyclase, phospholipase C, and mitogen-activated protein kinases. The binding of this compound to the adenosine A3 receptor inhibits adenylate cyclase activity, leading to a decrease in cyclic adenosine monophosphate levels. Additionally, this compound activates phospholipase C, resulting in the production of inositol triphosphate and diacylglycerol, which are important secondary messengers in cellular signaling pathways .
Cellular Effects
This compound exerts various effects on different types of cells and cellular processes. In immune cells, such as macrophages and dendritic cells, this compound inhibits the production of pro-inflammatory cytokines, including tumor necrosis factor-alpha and interleukin-6. This anti-inflammatory effect is mediated through the activation of the adenosine A3 receptor, which leads to the suppression of nuclear factor-kappa B signaling pathways. In cancer cells, this compound induces apoptosis and inhibits cell proliferation by modulating the expression of genes involved in cell cycle regulation and apoptosis. Furthermore, this compound influences cellular metabolism by altering the activity of key metabolic enzymes and pathways .
Molecular Mechanism
The molecular mechanism of action of this compound involves its binding to the adenosine A3 receptor, which triggers a cascade of intracellular signaling events. Upon binding, this compound induces a conformational change in the receptor, leading to the activation of G proteins. This activation results in the inhibition of adenylate cyclase and the activation of phospholipase C. The downstream effects of these signaling events include changes in cyclic adenosine monophosphate levels, calcium mobilization, and the activation of protein kinase C. Additionally, this compound modulates gene expression by influencing transcription factors, such as nuclear factor-kappa B and activator protein-1 .
Temporal Effects in Laboratory Settings
In laboratory settings, the effects of this compound have been observed to change over time. The stability of this compound is influenced by factors such as temperature, pH, and the presence of other biomolecules. Studies have shown that this compound is relatively stable at physiological pH and temperature, but it can degrade over time in the presence of certain enzymes. Long-term exposure to this compound has been associated with sustained anti-inflammatory and anti-proliferative effects in various cell types. Prolonged exposure may also lead to desensitization of the adenosine A3 receptor, resulting in reduced efficacy of the compound .
Dosage Effects in Animal Models
The effects of this compound vary with different dosages in animal models. At low doses, this compound has been shown to exert anti-inflammatory and cardioprotective effects without significant toxicity. At higher doses, this compound can induce adverse effects, such as hypotension and bradycardia, due to its potent agonistic activity on the adenosine A3 receptor. Threshold effects have been observed, where a minimum effective dose is required to achieve therapeutic benefits, while doses above this threshold may lead to toxicity .
Metabolic Pathways
This compound is involved in several metabolic pathways, including those related to adenosine metabolism and signal transduction. It interacts with enzymes such as adenosine deaminase and adenosine kinase, which are involved in the regulation of adenosine levels in cells. This compound also affects metabolic flux by modulating the activity of key enzymes in glycolysis and oxidative phosphorylation. These interactions can lead to changes in metabolite levels and overall cellular energy balance .
Transport and Distribution
The transport and distribution of this compound within cells and tissues are mediated by specific transporters and binding proteins. This compound is taken up by cells through nucleoside transporters, which facilitate its entry into the cytoplasm. Once inside the cell, this compound can bind to intracellular proteins, such as heat shock proteins and chaperones, which influence its localization and accumulation. The distribution of this compound within tissues is influenced by factors such as blood flow, tissue permeability, and the presence of binding sites .
Subcellular Localization
The subcellular localization of this compound is critical for its activity and function. This compound is primarily localized in the cytoplasm, where it interacts with the adenosine A3 receptor on the cell membrane. Additionally, this compound can be found in other cellular compartments, such as the endoplasmic reticulum and mitochondria, where it may exert additional effects. The targeting of this compound to specific subcellular compartments is influenced by post-translational modifications and the presence of targeting signals .
Preparation Methods
Synthetic Routes and Reaction Conditions: The synthesis of N6-(4-Aminobenzyl)-9-[5-(methylcarbonyl)-β-D-ribofuranosyl]adenine involves several key steps:
Formation of the Ribofuranosyl Moiety: The ribofuranosyl group is introduced through a glycosylation reaction.
Aminobenzylation: The introduction of the 4-aminobenzyl group is achieved via a nucleophilic substitution reaction.
Methylcarbonylation: The methylcarbonyl group is added through an acylation reaction.
Industrial Production Methods: Industrial production of N6-(4-Aminobenzyl)-9-[5-(methylcarbonyl)-β-D-ribofuranosyl]adenine typically involves large-scale synthesis using optimized reaction conditions to ensure high yield and purity. The process includes:
Batch Processing: Utilizing large reactors to carry out the glycosylation, nucleophilic substitution, and acylation reactions.
Purification: Employing techniques such as crystallization and chromatography to purify the final product.
Types of Reactions:
Oxidation: N6-(4-Aminobenzyl)-9-[5-(methylcarbonyl)-β-D-ribofuranosyl]adenine can undergo oxidation reactions, particularly at the aminobenzyl group.
Reduction: The compound can be reduced to form various derivatives, depending on the reducing agents used.
Substitution: Nucleophilic substitution reactions can occur at the ribofuranosyl moiety.
Common Reagents and Conditions:
Oxidation: Common oxidizing agents include hydrogen peroxide and potassium permanganate.
Reduction: Reducing agents such as sodium borohydride and lithium aluminum hydride are used.
Substitution: Nucleophiles like amines and thiols are employed under basic conditions.
Major Products:
Oxidation Products: Oxidized derivatives of the aminobenzyl group.
Reduction Products: Reduced forms of the ribofuranosyl moiety.
Substitution Products: Substituted derivatives at the ribofuranosyl position.
Scientific Research Applications
N6-(4-Aminobenzyl)-9-[5-(methylcarbonyl)-β-D-ribofuranosyl]adenine has a wide range of applications in scientific research:
Chemistry: Used as a tool to study the binding and activity of adenosine receptors.
Biology: Investigates the role of adenosine receptors in various biological processes, including inflammation and immune response.
Medicine: Explores potential therapeutic applications in conditions such as asthma, cancer, and cardiovascular diseases.
Industry: Utilized in the development of diagnostic tools and pharmaceutical formulations
Comparison with Similar Compounds
N6-(4-Aminobenzyl)-9-[5-(methylcarbonyl)-β-D-ribofuranosyl]adenine is unique due to its high affinity and selectivity for the A3 adenosine receptor. Similar compounds include:
N6-(4-Amino-3-iodobenzyl)adenosine-5’-N-methylcarboxamide (I-AB-MECA): A radiolabeled derivative used in binding assays.
N6-(2-Phenylisopropyl)adenosine (PIA): Another adenosine receptor agonist with different selectivity profiles
N6-(4-Aminobenzyl)-9-[5-(methylcarbonyl)-β-D-ribofuranosyl]adenine stands out for its specific applications in research and potential therapeutic uses.
Properties
IUPAC Name |
(2S,3S,4R,5R)-5-[6-[(4-aminophenyl)methylamino]purin-9-yl]-3,4-dihydroxy-N-methyloxolane-2-carboxamide | |
---|---|---|
Source | PubChem | |
URL | https://pubchem.ncbi.nlm.nih.gov | |
Description | Data deposited in or computed by PubChem | |
InChI |
InChI=1S/C18H21N7O4/c1-20-17(28)14-12(26)13(27)18(29-14)25-8-24-11-15(22-7-23-16(11)25)21-6-9-2-4-10(19)5-3-9/h2-5,7-8,12-14,18,26-27H,6,19H2,1H3,(H,20,28)(H,21,22,23)/t12-,13+,14-,18+/m0/s1 | |
Source | PubChem | |
URL | https://pubchem.ncbi.nlm.nih.gov | |
Description | Data deposited in or computed by PubChem | |
InChI Key |
LDYMCRRFCMRFKB-MOROJQBDSA-N | |
Source | PubChem | |
URL | https://pubchem.ncbi.nlm.nih.gov | |
Description | Data deposited in or computed by PubChem | |
Canonical SMILES |
CNC(=O)C1C(C(C(O1)N2C=NC3=C(N=CN=C32)NCC4=CC=C(C=C4)N)O)O | |
Source | PubChem | |
URL | https://pubchem.ncbi.nlm.nih.gov | |
Description | Data deposited in or computed by PubChem | |
Isomeric SMILES |
CNC(=O)[C@@H]1[C@H]([C@H]([C@@H](O1)N2C=NC3=C(N=CN=C32)NCC4=CC=C(C=C4)N)O)O | |
Source | PubChem | |
URL | https://pubchem.ncbi.nlm.nih.gov | |
Description | Data deposited in or computed by PubChem | |
Molecular Formula |
C18H21N7O4 | |
Source | PubChem | |
URL | https://pubchem.ncbi.nlm.nih.gov | |
Description | Data deposited in or computed by PubChem | |
DSSTOX Substance ID |
DTXSID70415501 | |
Record name | 5-[6-[(4-Aminophenyl)methylamino]purin-9-yl]-3,4-dihydroxy-N-methyloxolane-2-carboxamide | |
Source | EPA DSSTox | |
URL | https://comptox.epa.gov/dashboard/DTXSID70415501 | |
Description | DSSTox provides a high quality public chemistry resource for supporting improved predictive toxicology. | |
Molecular Weight |
399.4 g/mol | |
Source | PubChem | |
URL | https://pubchem.ncbi.nlm.nih.gov | |
Description | Data deposited in or computed by PubChem | |
CAS No. |
152918-26-8 | |
Record name | 5-[6-[(4-Aminophenyl)methylamino]purin-9-yl]-3,4-dihydroxy-N-methyloxolane-2-carboxamide | |
Source | EPA DSSTox | |
URL | https://comptox.epa.gov/dashboard/DTXSID70415501 | |
Description | DSSTox provides a high quality public chemistry resource for supporting improved predictive toxicology. | |
Retrosynthesis Analysis
AI-Powered Synthesis Planning: Our tool employs the Template_relevance Pistachio, Template_relevance Bkms_metabolic, Template_relevance Pistachio_ringbreaker, Template_relevance Reaxys, Template_relevance Reaxys_biocatalysis model, leveraging a vast database of chemical reactions to predict feasible synthetic routes.
One-Step Synthesis Focus: Specifically designed for one-step synthesis, it provides concise and direct routes for your target compounds, streamlining the synthesis process.
Accurate Predictions: Utilizing the extensive PISTACHIO, BKMS_METABOLIC, PISTACHIO_RINGBREAKER, REAXYS, REAXYS_BIOCATALYSIS database, our tool offers high-accuracy predictions, reflecting the latest in chemical research and data.
Strategy Settings
Precursor scoring | Relevance Heuristic |
---|---|
Min. plausibility | 0.01 |
Model | Template_relevance |
Template Set | Pistachio/Bkms_metabolic/Pistachio_ringbreaker/Reaxys/Reaxys_biocatalysis |
Top-N result to add to graph | 6 |
Feasible Synthetic Routes
Disclaimer and Information on In-Vitro Research Products
Please be aware that all articles and product information presented on BenchChem are intended solely for informational purposes. The products available for purchase on BenchChem are specifically designed for in-vitro studies, which are conducted outside of living organisms. In-vitro studies, derived from the Latin term "in glass," involve experiments performed in controlled laboratory settings using cells or tissues. It is important to note that these products are not categorized as medicines or drugs, and they have not received approval from the FDA for the prevention, treatment, or cure of any medical condition, ailment, or disease. We must emphasize that any form of bodily introduction of these products into humans or animals is strictly prohibited by law. It is essential to adhere to these guidelines to ensure compliance with legal and ethical standards in research and experimentation.