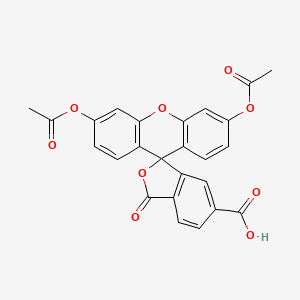
6-Carboxyfluorescein diacetate
Overview
Description
6-Carboxyfluorescein diacetate (6-CFDA) is a cell-permeant fluorogenic dye widely used in biological research to assess cell viability, membrane integrity, and intracellular enzymatic activity. The compound is non-fluorescent in its diacetate form but becomes highly fluorescent upon hydrolysis by intracellular esterases, which cleave the acetate groups to release the membrane-impermeant 6-carboxyfluorescein (6-CF, λex/λem ≈ 492/517 nm) . This property makes 6-CFDA a critical tool for:
- Viability assays: Differentiating live (fluorescent green) from dead cells when paired with propidium iodide (PI) .
- Apoptosis detection: Identifying early apoptotic cells via annexin V-Cy3 co-staining .
- Cell tracking: Monitoring clonal expansion in T-cell differentiation studies .
- pH measurement: Quantifying vacuolar and cytosolic pH in yeast and hepatocytes .
Its molecular weight is 460 g/mol (C25H16O9), and it is typically dissolved in dimethyl sulfoxide (DMSO) for experimental use .
Preparation Methods
Synthetic Routes to 6-Carboxyfluorescein Diacetate
Acetylation of 5(6)-Carboxyfluorescein
The foundational step in 6-CFDA synthesis involves diacetylation of 5(6)-carboxyfluorescein. A scalable protocol reported by Bradley et al. () utilizes acetic anhydride (180 mL) and pyridine (18 mL) at 110°C for 30 minutes, achieving 98% conversion (15 g scale). This exothermic reaction proceeds via nucleophilic acyl substitution, protecting phenolic hydroxyl groups while leaving the carboxylic acid moiety intact. Critical to reproducibility is the exclusion of moisture, as hydrolysis competes with acetylation. Post-reaction workup includes dissolution in ethyl acetate (300 mL), washing with 1 M potassium bisulfate (2 × 300 mL), and brine (300 mL), followed by sodium sulfate drying and vacuum concentration.
An alternative precursor synthesis route () employs 1,2,4-benzenetricarboxylic anhydride (9 g, 46.84 mmol) and resorcinol (10.32 g, 93.69 mmol) catalyzed by zinc bromide (15.82 g, 70.27 mmol) at 180°C for 80 minutes. This Friedel-Crafts acylation forms carboxyfluorescein, which is subsequently refluxed in 1 M HCl (700 mL) to hydrolyze byproducts. Filtration and vacuum drying yield 5(6)-carboxyfluorescein, which serves as the substrate for diacetylation.
Regioselective Isolation of the 6-Isomer
Separation of 5- and this compound isomers remains a key challenge due to their structural similarity. The Edinburgh group () developed a silica gel chromatography method using ethyl acetate/toluene (20:80 v/v), achieving baseline resolution (Rf = 0.53 for 6-isomer). This solvent system exploits differential polarity between isomers, with the 6-carboxy derivative eluting faster due to reduced hydrogen bonding capacity. At 15 g starting material scale, the process yields 25% pure 6-CFDA, contaminated with <1% 5-isomer.
Large-scale industrial protocols (e.g., Biotium) employ fractional crystallization from dimethyl sulfoxide (DMSO)/water mixtures. Cooling saturated DMSO solutions to 4°C preferentially precipitates the 6-isomer, though yields are lower (≈18%) compared to chromatographic methods.
Reaction Optimization and Kinetic Analysis
Catalytic Systems
Comparative studies ( vs.) reveal zinc bromide catalysis () increases carboxyfluorescein precursor purity (92% vs. 85% with HCl alone) but introduces heavy metal contamination risks. Pyridine/acetic anhydride systems () avoid metallic catalysts, making them preferable for biomedical applications. Kinetic profiling shows pseudo-first-order behavior for diacetylation (k = 0.12 min⁻¹ at 110°C), with an activation energy of 72.4 kJ/mol derived from Arrhenius plots.
Solvent Effects
Polar aprotic solvents (DMF, DMSO) accelerate acetylation but promote ester hydrolysis. Dichloromethane (DCM), used in N-hydroxysuccinimide (NHS) ester derivatization (), minimizes hydrolysis but requires extended reaction times (24 hours for 90% conversion). Optimal solvent selection balances reaction rate and product stability, with acetic anhydride doubling as both reagent and solvent in industrial settings.
Analytical Validation of 6-CFDA
Chromatographic Purity Assessment
HPLC analysis () using a C18 column (4.6 × 50 mm) and acetonitrile/water gradient (5–95% over 10 min) resolves 5- and 6-isomers at 7.5 and 7.8 minutes, respectively. UV detection at 495 nm confirms >99% purity post-chromatography, with molar extinction coefficients (ε) of 78,000 M⁻¹cm⁻¹ for both isomers.
Spectroscopic Characterization
¹H NMR (500 MHz, DMSO-d6) of 6-CFDA exhibits distinct resonances: δ 8.15 (d, J = 8.5 Hz, 1H, aromatic), 7.25 (s, 1H, xanthene), 2.35 (s, 6H, acetate methyl). Carbonyl carbons appear at δ 169.8 (acetate) and 172.4 (carboxylic acid) in ¹³C NMR, confirming successful diacetylation.
Table 1: Comparative Synthesis Methods for this compound
Industrial-Scale Production Considerations
Cost-Benefit Analysis
Chromatography, while high-yielding (25%), requires substantial solvent volumes (≈10 L/kg product), increasing production costs. Crystallization methods reduce solvent use but necessitate DMSO recycling systems. Biotium’s patented process () combines acetylation in acetic anhydride with in-situ crystallization, achieving 85% yield at pilot scale (50 kg batches).
Regulatory Compliance
Good Manufacturing Practice (GMP) guidelines mandate residual pyridine levels <50 ppm in final products. Post-synthesis treatments include activated carbon filtration (0.5% w/w) and azeotropic distillation with toluene to meet pharmacopeial standards.
Chemical Reactions Analysis
Types of Reactions
6-Carboxyfluorescein diacetate undergoes several types of chemical reactions, including:
Hydrolysis: The ester bonds are hydrolyzed by intracellular esterases, converting the compound into 6-carboxyfluorescein.
Oxidation and Reduction: The compound can participate in redox reactions, although these are less common in biological applications.
Common Reagents and Conditions
Hydrolysis: Typically occurs in aqueous environments within cells, facilitated by esterases.
Oxidation and Reduction: These reactions may require specific oxidizing or reducing agents under controlled conditions.
Major Products Formed
Scientific Research Applications
Chemical Properties and Mechanism of Action
6-Carboxyfluorescein diacetate is a membrane-permeable compound that, upon entering cells, is hydrolyzed by intracellular esterases to release the fluorescent 6-carboxyfluorescein. This property allows it to be employed effectively for labeling live cells, facilitating real-time observation of cellular activities.
Key Applications
-
Cell Proliferation Studies
- This compound is extensively used to analyze T lymphocyte proliferation in various settings. For instance, studies have demonstrated its effectiveness in assessing mitogenic responses in patients with primary immunodeficiencies, where it helped distinguish between normal and impaired T-cell responses .
-
Immunological Assays
- The compound is employed in flow cytometry for labeling cells to evaluate immune responses. It has been shown to enhance the detection of endothelial progenitor cells in human peripheral blood, providing a reliable method for quantifying these cells using CFSE-labeled microspheres as reference standards .
- Cancer Research
- Cell Tracking
Case Study 1: T Lymphocyte Proliferation
A study involving 46 unclassified patients used this compound to evaluate T-cell disorders. Results indicated significantly lower CD3+ blast cell counts after stimulation compared to healthy controls, highlighting the dye's role in diagnosing immunodeficiencies .
Case Study 2: Endothelial Progenitor Cells Detection
Research established a single-platform flow cytometry method using this compound-labeled microspheres for detecting endothelial progenitor cells. This method proved faster and more cost-effective than traditional techniques while maintaining accuracy in cell quantification .
Data Table: Summary of Applications
Mechanism of Action
The mechanism of action of 6-Carboxyfluorescein diacetate involves its uptake by cells, where intracellular esterases hydrolyze the ester bonds, converting it into 6-carboxyfluorescein. This conversion results in a highly fluorescent compound that can be detected using various fluorescence-based techniques. The fluorescence intensity decreases with each cell division, allowing researchers to track cell proliferation .
Comparison with Similar Compounds
Comparative Analysis with Structural Analogs
5-Carboxyfluorescein Diacetate (5-CFDA)
5-CFDA is a positional isomer of 6-CFDA, differing only in the carboxyl group’s position on the fluorescein core. Key distinctions include:
- Synthetic separation : The diacetate protection of 5(6)-carboxyfluorescein followed by N-hydroxysuccinimide (NHS) ester synthesis allows gram-scale separation of 5-CFDA and 6-CFDA .
- Fluorescence properties : Both isomers exhibit similar excitation/emission profiles but may differ in quantum yield due to steric effects.
- Applications : 5-CFDA is less commonly used in viability assays but is preferred in cell division tracking (e.g., T-cell proliferation studies using 5(6)-carboxyfluorescein succinimidyl ester) .
Property | 6-CFDA | 5-CFDA |
---|---|---|
Molecular Weight | 460 g/mol | 460 g/mol |
Fluorescence (λex/λem) | 492/517 nm | 492/517 nm |
Primary Applications | Viability, apoptosis, pH | Cell division tracking |
Key References |
5(6)-Carboxy-2',7'-Dichlorofluorescein Diacetate (CDCFDA)
CDCFDA incorporates chlorine substitutions at the 2' and 7' positions of the fluorescein core, conferring distinct advantages:
- Lower pKa : The dichloro modification reduces the pKa from ~6.3 (6-CF) to ~4.8, making CDCFDA suitable for acidic environments (e.g., lysosomal pH measurement) .
- Enhanced photostability : Chlorine substitutions improve resistance to photobleaching.
- Applications : CDCFDA is ideal for low-pH compartments and dual-labeling experiments with other fluorophores .
Carboxyrhodamine B Derivatives
Carboxyrhodamine B (CRB) and its diacetate forms (e.g., 5/6-carboxyrhodamine B diacetate) differ from 6-CFDA in their fluorescence profiles and chemical stability:
- Red-shifted emission : CRB derivatives emit in the orange-red range (λem ≈ 575 nm), enabling multiplexing with green fluorophores like 6-CFDA .
- Hydrophobicity : CRB’s structure enhances membrane retention, reducing dye leakage in long-term assays .
Cell Viability and Apoptosis
6-CFDA is the gold standard for viability assays, often paired with PI to distinguish live (green), apoptotic (green + red), and necrotic (red) cells . In hepatocytes, 6-CFDA processing into 6-CF and its secretion into bile canaliculi has been used to study polarity defects in LKB1-deficient mice .
pH Sensing
6-CFDA-derived 6-CF has been used in yeast vacuolar pH measurement (pH ~6.1–6.2) via dual-excitation flow cytometry , while CDCFDA is better suited for acidic organelles.
Biological Activity
6-Carboxyfluorescein diacetate (6-CFDA) is a fluorescent probe widely used in biological research to assess cell viability, proliferation, and metabolic activity. This compound is particularly notable for its ability to penetrate cell membranes and undergo hydrolysis by intracellular esterases, resulting in the release of the fluorescent 6-carboxyfluorescein (6-CF), which can be detected and quantified. This article explores the biological activity of 6-CFDA, including its mechanisms of action, applications in various studies, and relevant case studies.
6-CFDA is a membrane-permeant compound that, upon entering cells, is hydrolyzed by esterases to produce 6-carboxyfluorescein. This process can be summarized as follows:
- Uptake : 6-CFDA diffuses across the cell membrane.
- Hydrolysis : Inside the cell, esterases cleave the acetate groups, converting 6-CFDA into 6-CF.
- Fluorescence : The released 6-CF exhibits strong fluorescence, allowing for detection via fluorescence microscopy or flow cytometry.
Applications in Research
The biological activity of 6-CFDA has been leveraged in various research contexts:
- Cell Proliferation Studies : It is commonly used to assess T lymphocyte proliferation in immunological studies. For instance, a study demonstrated that 6-CFDA could effectively measure mitogenic responses in patients with primary immunodeficiencies .
- Metabolic Activity Assessment : Research has utilized 6-CFDA to evaluate metabolic processes in hepatocytes, providing insights into xenobiotic metabolism and cellular heterogeneity .
- Bacterial Activity Detection : While studies have shown that carboxyfluorescein diacetate (CFDA) can indicate bacterial activity, it was found that CFDA/SE (a derivative) was less effective due to non-specific labeling .
Case Studies
-
T Lymphocyte Proliferation :
- Study Overview : A study involving 46 unclassified patients assessed T-cell disorders using a CFSE-based assay. The results indicated significantly lower CD3+ blast cells after stimulation compared to healthy controls .
- Findings : The proliferation response was notably impaired in patients with combined immunodeficiencies, highlighting the utility of 6-CFDA in diagnosing T-cell defects.
-
Hepatocyte Metabolism :
- Study Overview : Researchers employed multiphoton fluorescence microscopy to study the metabolism of xenobiotics in hepatocytes using 6-CFDA .
- Findings : The study revealed distinct kinetics in the accumulation of fluorescence between the cytoplasm and nucleus, suggesting variations in drug transport mechanisms.
-
Bacterial Viability Testing :
- Study Overview : A comparative analysis evaluated the effectiveness of CFDA against other fluorescent probes for detecting bacterial viability .
- Findings : The study concluded that while CFDA was effective for detecting active bacteria, CFDA/SE failed to differentiate between active and inactive cells.
Research Findings Summary
Q & A
Basic Research Questions
Q. How does 6-CFDA function as a viability probe in cell-based assays?
6-CFDA is a cell-permeant, non-fluorescent compound that undergoes hydrolysis by intracellular esterases in metabolically active cells, converting it into fluorescent 6-carboxyfluorescein (6-CF). This fluorescence is retained in viable cells, while dead or necrotic cells (with compromised membranes) fail to process the dye. For quantitative analysis, 6-CFDA is often paired with propidium iodide (PI) or annexin V-Cy3 to differentiate viable, apoptotic, and necrotic populations .
Q. What experimental steps are required to differentiate apoptotic, necrotic, and viable cells using 6-CFDA?
- Protocol :
- Incubate cells with 6-CFDA (10–20 µM, 15–30 min at 37°C) and annexin V-Cy3 (per kit instructions).
- Wash cells to remove excess dye.
- Analyze via fluorescence microscopy or flow cytometry:
- Viable : Green fluorescence (6-CF only).
- Apoptotic : Green + red fluorescence (6-CF + annexin V-Cy3).
- Necrotic : Red fluorescence (annexin V-Cy3 only) .
Q. What are the spectral properties of 6-CFDA and its hydrolyzed product?
- 6-CFDA (non-fluorescent) : Requires esterase cleavage.
- 6-CF (fluorescent) :
Advanced Research Questions
Q. How can researchers optimize 6-CFDA staining protocols for heterogeneous cell populations?
- Key variables :
- Concentration : Titrate between 5–50 µM to avoid overloading low-activity cells (e.g., encapsulated islet cells ).
- Incubation time : Adjust based on cell metabolic rates (e.g., 20 min for hepatocytes vs. 45 min for fungal hyphae ).
- Validation : Use PI or trypan blue as a counterstain for dead cells .
Q. What are the technical considerations when combining 6-CFDA with other fluorescent probes (e.g., annexin V-Cy3) to avoid spectral overlap?
- Spectral separation :
Q. How does 6-CFDA-based viability assessment compare to alternative methods like propidium iodide (PI) exclusion in encapsulated cell systems?
- Advantages of 6-CFDA : Labels live cells proactively, enabling real-time tracking.
- PI limitation : Only labels dead cells post-membrane rupture.
- Example : In microencapsulated fetal pig islet cells, 6-CFDA/PI co-staining provided dynamic viability data over 72 hours .
Q. What methodologies enable real-time tracking of 6-CFDA processing in vivo, such as hepatobiliary excretion studies?
- Intravital multiphoton microscopy :
Q. How can researchers address challenges with low fluorescence signal intensity in 6-CFDA assays?
- Solutions :
- Increase dye concentration (up to 50 µM) or incubation time.
- Validate esterase activity with a positive control (e.g., untreated cells).
- Avoid prolonged light exposure to prevent photobleaching .
Q. What are the implications of cell-type-specific esterase activity variations on 6-CFDA data interpretation?
- Critical consideration : Low esterase activity (e.g., quiescent lymphocytes) may yield false negatives.
- Mitigation : Pre-test cells with a range of 6-CFDA concentrations and validate with ATP-based assays .
Q. How does 6-CFDA facilitate the study of solute transport in specialized cellular systems, such as fungal hyphae or plant embryos?
Properties
IUPAC Name |
3',6'-diacetyloxy-1-oxospiro[2-benzofuran-3,9'-xanthene]-5-carboxylic acid | |
---|---|---|
Source | PubChem | |
URL | https://pubchem.ncbi.nlm.nih.gov | |
Description | Data deposited in or computed by PubChem | |
InChI |
InChI=1S/C25H16O9/c1-12(26)31-15-4-7-18-21(10-15)33-22-11-16(32-13(2)27)5-8-19(22)25(18)20-9-14(23(28)29)3-6-17(20)24(30)34-25/h3-11H,1-2H3,(H,28,29) | |
Source | PubChem | |
URL | https://pubchem.ncbi.nlm.nih.gov | |
Description | Data deposited in or computed by PubChem | |
InChI Key |
QMOGCCYGOPYYNT-UHFFFAOYSA-N | |
Source | PubChem | |
URL | https://pubchem.ncbi.nlm.nih.gov | |
Description | Data deposited in or computed by PubChem | |
Canonical SMILES |
CC(=O)OC1=CC2=C(C=C1)C3(C4=C(O2)C=C(C=C4)OC(=O)C)C5=C(C=CC(=C5)C(=O)O)C(=O)O3 | |
Source | PubChem | |
URL | https://pubchem.ncbi.nlm.nih.gov | |
Description | Data deposited in or computed by PubChem | |
Molecular Formula |
C25H16O9 | |
Source | PubChem | |
URL | https://pubchem.ncbi.nlm.nih.gov | |
Description | Data deposited in or computed by PubChem | |
DSSTOX Substance ID |
DTXSID80187121 | |
Record name | 6-Carboxyfluorescein diacetate | |
Source | EPA DSSTox | |
URL | https://comptox.epa.gov/dashboard/DTXSID80187121 | |
Description | DSSTox provides a high quality public chemistry resource for supporting improved predictive toxicology. | |
Molecular Weight |
460.4 g/mol | |
Source | PubChem | |
URL | https://pubchem.ncbi.nlm.nih.gov | |
Description | Data deposited in or computed by PubChem | |
CAS No. |
3348-03-6 | |
Record name | 6-Carboxyfluorescein diacetate | |
Source | ChemIDplus | |
URL | https://pubchem.ncbi.nlm.nih.gov/substance/?source=chemidplus&sourceid=0003348036 | |
Description | ChemIDplus is a free, web search system that provides access to the structure and nomenclature authority files used for the identification of chemical substances cited in National Library of Medicine (NLM) databases, including the TOXNET system. | |
Record name | 6-Carboxyfluorescein diacetate | |
Source | EPA DSSTox | |
URL | https://comptox.epa.gov/dashboard/DTXSID80187121 | |
Description | DSSTox provides a high quality public chemistry resource for supporting improved predictive toxicology. | |
Record name | 6-Carboxyfluorescein Diacetate | |
Source | European Chemicals Agency (ECHA) | |
URL | https://echa.europa.eu/information-on-chemicals | |
Description | The European Chemicals Agency (ECHA) is an agency of the European Union which is the driving force among regulatory authorities in implementing the EU's groundbreaking chemicals legislation for the benefit of human health and the environment as well as for innovation and competitiveness. | |
Explanation | Use of the information, documents and data from the ECHA website is subject to the terms and conditions of this Legal Notice, and subject to other binding limitations provided for under applicable law, the information, documents and data made available on the ECHA website may be reproduced, distributed and/or used, totally or in part, for non-commercial purposes provided that ECHA is acknowledged as the source: "Source: European Chemicals Agency, http://echa.europa.eu/". Such acknowledgement must be included in each copy of the material. ECHA permits and encourages organisations and individuals to create links to the ECHA website under the following cumulative conditions: Links can only be made to webpages that provide a link to the Legal Notice page. | |
Retrosynthesis Analysis
AI-Powered Synthesis Planning: Our tool employs the Template_relevance Pistachio, Template_relevance Bkms_metabolic, Template_relevance Pistachio_ringbreaker, Template_relevance Reaxys, Template_relevance Reaxys_biocatalysis model, leveraging a vast database of chemical reactions to predict feasible synthetic routes.
One-Step Synthesis Focus: Specifically designed for one-step synthesis, it provides concise and direct routes for your target compounds, streamlining the synthesis process.
Accurate Predictions: Utilizing the extensive PISTACHIO, BKMS_METABOLIC, PISTACHIO_RINGBREAKER, REAXYS, REAXYS_BIOCATALYSIS database, our tool offers high-accuracy predictions, reflecting the latest in chemical research and data.
Strategy Settings
Precursor scoring | Relevance Heuristic |
---|---|
Min. plausibility | 0.01 |
Model | Template_relevance |
Template Set | Pistachio/Bkms_metabolic/Pistachio_ringbreaker/Reaxys/Reaxys_biocatalysis |
Top-N result to add to graph | 6 |
Feasible Synthetic Routes
Disclaimer and Information on In-Vitro Research Products
Please be aware that all articles and product information presented on BenchChem are intended solely for informational purposes. The products available for purchase on BenchChem are specifically designed for in-vitro studies, which are conducted outside of living organisms. In-vitro studies, derived from the Latin term "in glass," involve experiments performed in controlled laboratory settings using cells or tissues. It is important to note that these products are not categorized as medicines or drugs, and they have not received approval from the FDA for the prevention, treatment, or cure of any medical condition, ailment, or disease. We must emphasize that any form of bodily introduction of these products into humans or animals is strictly prohibited by law. It is essential to adhere to these guidelines to ensure compliance with legal and ethical standards in research and experimentation.