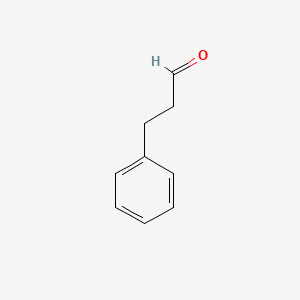
Hydrocinnamaldehyde
Overview
Description
Dihydrocinnamaldehyde, also known as 3-phenylpropionaldehyde, is an organic compound with the formula C₆H₅CH₂CH₂CHO. It is a colorless liquid that is produced by the hydrogenation of cinnamaldehyde. This compound is commonly used in organic synthesis and has various applications in different fields .
Preparation Methods
Synthetic Routes and Reaction Conditions
Dihydrocinnamaldehyde is typically synthesized through the selective hydrogenation of cinnamaldehyde. This process involves the use of catalysts such as palladium (Pd) nanoparticles deposited on nitrogen-doped mesoporous carbon (NMC). The reaction is carried out at room temperature (30°C) under low hydrogen pressure, resulting in high selectivity and conversion rates .
Industrial Production Methods
In industrial settings, dithis compound can be produced continuously by reacting benzaldehyde derivatives with alkanals in the presence of bases. This is followed by hydrogenation in a circulation reactor using a suspension catalyst and hydrogen .
Chemical Reactions Analysis
Types of Reactions
Dihydrocinnamaldehyde undergoes various chemical reactions, including:
Oxidation: It can be oxidized to form cinnamic acid or other related compounds.
Reduction: It can be further reduced to form hydrocinnamyl alcohol.
Substitution: It can participate in substitution reactions, particularly at the aldehyde group.
Common Reagents and Conditions
Oxidation: Common oxidizing agents include potassium permanganate (KMnO₄) and chromium trioxide (CrO₃).
Reduction: Catalysts such as palladium on carbon (Pd/C) and hydrogen gas (H₂) are used.
Substitution: Various nucleophiles can be used depending on the desired product.
Major Products
Oxidation: Cinnamic acid
Reduction: Hydrocinnamyl alcohol
Substitution: Various substituted phenylpropionaldehyde derivatives
Scientific Research Applications
Dihydrocinnamaldehyde has a wide range of applications in scientific research:
Chemistry: It is used as a substrate in organic synthesis and mechanistic studies.
Biology: It is studied for its potential biological activities and interactions with biomolecules.
Medicine: Research is ongoing to explore its potential therapeutic properties.
Industry: It is used in the fragrance industry to produce various aromatic compounds.
Mechanism of Action
The mechanism of action of dihydrocinnamaldehyde involves its interaction with molecular targets and pathways. For example, in hydrogenation reactions, the compound interacts with palladium nanoparticles, leading to the selective hydrogenation of the alkene subunit . The presence of nitrogen in the catalyst enhances the catalytic activity and selectivity.
Comparison with Similar Compounds
Similar Compounds
Cinnamaldehyde: The parent compound from which dihydrocinnamaldehyde is derived.
This compound: Another derivative produced by the hydrogenation of cinnamaldehyde.
α-Amylcinnamaldehyde: A commercial fragrance compound.
α-Hexylcinnamaldehyde: Another commercial fragrance compound.
Uniqueness
Dithis compound is unique due to its selective hydrogenation process, which allows for high conversion rates and selectivity. Its applications in various fields, including organic synthesis and the fragrance industry, highlight its versatility and importance.
Properties
IUPAC Name |
3-phenylpropanal | |
---|---|---|
Source | PubChem | |
URL | https://pubchem.ncbi.nlm.nih.gov | |
Description | Data deposited in or computed by PubChem | |
InChI |
InChI=1S/C9H10O/c10-8-4-7-9-5-2-1-3-6-9/h1-3,5-6,8H,4,7H2 | |
Source | PubChem | |
URL | https://pubchem.ncbi.nlm.nih.gov | |
Description | Data deposited in or computed by PubChem | |
InChI Key |
YGCZTXZTJXYWCO-UHFFFAOYSA-N | |
Source | PubChem | |
URL | https://pubchem.ncbi.nlm.nih.gov | |
Description | Data deposited in or computed by PubChem | |
Canonical SMILES |
C1=CC=C(C=C1)CCC=O | |
Source | PubChem | |
URL | https://pubchem.ncbi.nlm.nih.gov | |
Description | Data deposited in or computed by PubChem | |
Molecular Formula |
C9H10O | |
Source | PubChem | |
URL | https://pubchem.ncbi.nlm.nih.gov | |
Description | Data deposited in or computed by PubChem | |
DSSTOX Substance ID |
DTXSID0047610 | |
Record name | 3-Phenylpropanal | |
Source | EPA DSSTox | |
URL | https://comptox.epa.gov/dashboard/DTXSID0047610 | |
Description | DSSTox provides a high quality public chemistry resource for supporting improved predictive toxicology. | |
Molecular Weight |
134.17 g/mol | |
Source | PubChem | |
URL | https://pubchem.ncbi.nlm.nih.gov | |
Description | Data deposited in or computed by PubChem | |
Physical Description |
Colorless or light yellow liquid; [Acros Organics MSDS], Solid, Colourless to slightly yellow liquid, strong pungent floral odour of hyacinth | |
Record name | 3-Phenylpropionaldehyde | |
Source | Haz-Map, Information on Hazardous Chemicals and Occupational Diseases | |
URL | https://haz-map.com/Agents/21015 | |
Description | Haz-Map® is an occupational health database designed for health and safety professionals and for consumers seeking information about the adverse effects of workplace exposures to chemical and biological agents. | |
Explanation | Copyright (c) 2022 Haz-Map(R). All rights reserved. Unless otherwise indicated, all materials from Haz-Map are copyrighted by Haz-Map(R). No part of these materials, either text or image may be used for any purpose other than for personal use. Therefore, reproduction, modification, storage in a retrieval system or retransmission, in any form or by any means, electronic, mechanical or otherwise, for reasons other than personal use, is strictly prohibited without prior written permission. | |
Record name | 3-Phenylpropanal | |
Source | Human Metabolome Database (HMDB) | |
URL | http://www.hmdb.ca/metabolites/HMDB0033716 | |
Description | The Human Metabolome Database (HMDB) is a freely available electronic database containing detailed information about small molecule metabolites found in the human body. | |
Explanation | HMDB is offered to the public as a freely available resource. Use and re-distribution of the data, in whole or in part, for commercial purposes requires explicit permission of the authors and explicit acknowledgment of the source material (HMDB) and the original publication (see the HMDB citing page). We ask that users who download significant portions of the database cite the HMDB paper in any resulting publications. | |
Record name | 3-Phenylpropionaldehyde | |
Source | Joint FAO/WHO Expert Committee on Food Additives (JECFA) | |
URL | https://www.fao.org/food/food-safety-quality/scientific-advice/jecfa/jecfa-flav/details/en/c/689/ | |
Description | The flavoring agent databse provides the most recent specifications for flavorings evaluated by JECFA. | |
Explanation | Permission from WHO is not required for the use of WHO materials issued under the Creative Commons Attribution-NonCommercial-ShareAlike 3.0 Intergovernmental Organization (CC BY-NC-SA 3.0 IGO) licence. | |
Boiling Point |
97.00 to 98.00 °C. @ 12.00 mm Hg | |
Record name | 3-Phenylpropanal | |
Source | Human Metabolome Database (HMDB) | |
URL | http://www.hmdb.ca/metabolites/HMDB0033716 | |
Description | The Human Metabolome Database (HMDB) is a freely available electronic database containing detailed information about small molecule metabolites found in the human body. | |
Explanation | HMDB is offered to the public as a freely available resource. Use and re-distribution of the data, in whole or in part, for commercial purposes requires explicit permission of the authors and explicit acknowledgment of the source material (HMDB) and the original publication (see the HMDB citing page). We ask that users who download significant portions of the database cite the HMDB paper in any resulting publications. | |
Solubility |
insoluble in water; soluble in oils, miscible (in ethanol) | |
Record name | 3-Phenylpropionaldehyde | |
Source | Joint FAO/WHO Expert Committee on Food Additives (JECFA) | |
URL | https://www.fao.org/food/food-safety-quality/scientific-advice/jecfa/jecfa-flav/details/en/c/689/ | |
Description | The flavoring agent databse provides the most recent specifications for flavorings evaluated by JECFA. | |
Explanation | Permission from WHO is not required for the use of WHO materials issued under the Creative Commons Attribution-NonCommercial-ShareAlike 3.0 Intergovernmental Organization (CC BY-NC-SA 3.0 IGO) licence. | |
Density |
1.008-1.018 | |
Record name | 3-Phenylpropionaldehyde | |
Source | Joint FAO/WHO Expert Committee on Food Additives (JECFA) | |
URL | https://www.fao.org/food/food-safety-quality/scientific-advice/jecfa/jecfa-flav/details/en/c/689/ | |
Description | The flavoring agent databse provides the most recent specifications for flavorings evaluated by JECFA. | |
Explanation | Permission from WHO is not required for the use of WHO materials issued under the Creative Commons Attribution-NonCommercial-ShareAlike 3.0 Intergovernmental Organization (CC BY-NC-SA 3.0 IGO) licence. | |
CAS No. |
104-53-0, 1335-10-0 | |
Record name | Benzenepropanal | |
Source | CAS Common Chemistry | |
URL | https://commonchemistry.cas.org/detail?cas_rn=104-53-0 | |
Description | CAS Common Chemistry is an open community resource for accessing chemical information. Nearly 500,000 chemical substances from CAS REGISTRY cover areas of community interest, including common and frequently regulated chemicals, and those relevant to high school and undergraduate chemistry classes. This chemical information, curated by our expert scientists, is provided in alignment with our mission as a division of the American Chemical Society. | |
Explanation | The data from CAS Common Chemistry is provided under a CC-BY-NC 4.0 license, unless otherwise stated. | |
Record name | 3-Phenylpropanal | |
Source | ChemIDplus | |
URL | https://pubchem.ncbi.nlm.nih.gov/substance/?source=chemidplus&sourceid=0000104530 | |
Description | ChemIDplus is a free, web search system that provides access to the structure and nomenclature authority files used for the identification of chemical substances cited in National Library of Medicine (NLM) databases, including the TOXNET system. | |
Record name | Propanal, phenyl- | |
Source | ChemIDplus | |
URL | https://pubchem.ncbi.nlm.nih.gov/substance/?source=chemidplus&sourceid=0001335100 | |
Description | ChemIDplus is a free, web search system that provides access to the structure and nomenclature authority files used for the identification of chemical substances cited in National Library of Medicine (NLM) databases, including the TOXNET system. | |
Record name | 3-Phenylpropanal | |
Source | DTP/NCI | |
URL | https://dtp.cancer.gov/dtpstandard/servlet/dwindex?searchtype=NSC&outputformat=html&searchlist=9271 | |
Description | The NCI Development Therapeutics Program (DTP) provides services and resources to the academic and private-sector research communities worldwide to facilitate the discovery and development of new cancer therapeutic agents. | |
Explanation | Unless otherwise indicated, all text within NCI products is free of copyright and may be reused without our permission. Credit the National Cancer Institute as the source. | |
Record name | Benzenepropanal | |
Source | EPA Chemicals under the TSCA | |
URL | https://www.epa.gov/chemicals-under-tsca | |
Description | EPA Chemicals under the Toxic Substances Control Act (TSCA) collection contains information on chemicals and their regulations under TSCA, including non-confidential content from the TSCA Chemical Substance Inventory and Chemical Data Reporting. | |
Record name | Propanal, phenyl- | |
Source | EPA Chemicals under the TSCA | |
URL | https://www.epa.gov/chemicals-under-tsca | |
Description | EPA Chemicals under the Toxic Substances Control Act (TSCA) collection contains information on chemicals and their regulations under TSCA, including non-confidential content from the TSCA Chemical Substance Inventory and Chemical Data Reporting. | |
Record name | 3-Phenylpropanal | |
Source | EPA DSSTox | |
URL | https://comptox.epa.gov/dashboard/DTXSID0047610 | |
Description | DSSTox provides a high quality public chemistry resource for supporting improved predictive toxicology. | |
Record name | Phenylpropionaldehyde | |
Source | European Chemicals Agency (ECHA) | |
URL | https://echa.europa.eu/substance-information/-/substanceinfo/100.014.201 | |
Description | The European Chemicals Agency (ECHA) is an agency of the European Union which is the driving force among regulatory authorities in implementing the EU's groundbreaking chemicals legislation for the benefit of human health and the environment as well as for innovation and competitiveness. | |
Explanation | Use of the information, documents and data from the ECHA website is subject to the terms and conditions of this Legal Notice, and subject to other binding limitations provided for under applicable law, the information, documents and data made available on the ECHA website may be reproduced, distributed and/or used, totally or in part, for non-commercial purposes provided that ECHA is acknowledged as the source: "Source: European Chemicals Agency, http://echa.europa.eu/". Such acknowledgement must be included in each copy of the material. ECHA permits and encourages organisations and individuals to create links to the ECHA website under the following cumulative conditions: Links can only be made to webpages that provide a link to the Legal Notice page. | |
Record name | 3-phenylpropionaldehyde | |
Source | European Chemicals Agency (ECHA) | |
URL | https://echa.europa.eu/substance-information/-/substanceinfo/100.002.920 | |
Description | The European Chemicals Agency (ECHA) is an agency of the European Union which is the driving force among regulatory authorities in implementing the EU's groundbreaking chemicals legislation for the benefit of human health and the environment as well as for innovation and competitiveness. | |
Explanation | Use of the information, documents and data from the ECHA website is subject to the terms and conditions of this Legal Notice, and subject to other binding limitations provided for under applicable law, the information, documents and data made available on the ECHA website may be reproduced, distributed and/or used, totally or in part, for non-commercial purposes provided that ECHA is acknowledged as the source: "Source: European Chemicals Agency, http://echa.europa.eu/". Such acknowledgement must be included in each copy of the material. ECHA permits and encourages organisations and individuals to create links to the ECHA website under the following cumulative conditions: Links can only be made to webpages that provide a link to the Legal Notice page. | |
Record name | 3-PHENYLPROPIONALDEHYDE | |
Source | FDA Global Substance Registration System (GSRS) | |
URL | https://gsrs.ncats.nih.gov/ginas/app/beta/substances/LP1E86N30T | |
Description | The FDA Global Substance Registration System (GSRS) enables the efficient and accurate exchange of information on what substances are in regulated products. Instead of relying on names, which vary across regulatory domains, countries, and regions, the GSRS knowledge base makes it possible for substances to be defined by standardized, scientific descriptions. | |
Explanation | Unless otherwise noted, the contents of the FDA website (www.fda.gov), both text and graphics, are not copyrighted. They are in the public domain and may be republished, reprinted and otherwise used freely by anyone without the need to obtain permission from FDA. Credit to the U.S. Food and Drug Administration as the source is appreciated but not required. | |
Record name | 3-Phenylpropanal | |
Source | Human Metabolome Database (HMDB) | |
URL | http://www.hmdb.ca/metabolites/HMDB0033716 | |
Description | The Human Metabolome Database (HMDB) is a freely available electronic database containing detailed information about small molecule metabolites found in the human body. | |
Explanation | HMDB is offered to the public as a freely available resource. Use and re-distribution of the data, in whole or in part, for commercial purposes requires explicit permission of the authors and explicit acknowledgment of the source material (HMDB) and the original publication (see the HMDB citing page). We ask that users who download significant portions of the database cite the HMDB paper in any resulting publications. | |
Melting Point |
47 °C | |
Record name | 3-Phenylpropanal | |
Source | Human Metabolome Database (HMDB) | |
URL | http://www.hmdb.ca/metabolites/HMDB0033716 | |
Description | The Human Metabolome Database (HMDB) is a freely available electronic database containing detailed information about small molecule metabolites found in the human body. | |
Explanation | HMDB is offered to the public as a freely available resource. Use and re-distribution of the data, in whole or in part, for commercial purposes requires explicit permission of the authors and explicit acknowledgment of the source material (HMDB) and the original publication (see the HMDB citing page). We ask that users who download significant portions of the database cite the HMDB paper in any resulting publications. | |
Synthesis routes and methods I
Procedure details
Synthesis routes and methods II
Procedure details
Synthesis routes and methods III
Procedure details
Synthesis routes and methods IV
Procedure details
Synthesis routes and methods V
Procedure details
Retrosynthesis Analysis
AI-Powered Synthesis Planning: Our tool employs the Template_relevance Pistachio, Template_relevance Bkms_metabolic, Template_relevance Pistachio_ringbreaker, Template_relevance Reaxys, Template_relevance Reaxys_biocatalysis model, leveraging a vast database of chemical reactions to predict feasible synthetic routes.
One-Step Synthesis Focus: Specifically designed for one-step synthesis, it provides concise and direct routes for your target compounds, streamlining the synthesis process.
Accurate Predictions: Utilizing the extensive PISTACHIO, BKMS_METABOLIC, PISTACHIO_RINGBREAKER, REAXYS, REAXYS_BIOCATALYSIS database, our tool offers high-accuracy predictions, reflecting the latest in chemical research and data.
Strategy Settings
Precursor scoring | Relevance Heuristic |
---|---|
Min. plausibility | 0.01 |
Model | Template_relevance |
Template Set | Pistachio/Bkms_metabolic/Pistachio_ringbreaker/Reaxys/Reaxys_biocatalysis |
Top-N result to add to graph | 6 |
Feasible Synthetic Routes
Q1: What is the molecular formula and weight of hydrocinnamaldehyde?
A1: this compound has the molecular formula C9H10O and a molecular weight of 134.17 g/mol.
Q2: Is there any spectroscopic data available to characterize this compound?
A2: Yes, several spectroscopic techniques have been used to characterize this compound. Researchers have used 13C labeling and mass spectrometry to study the electron-impact-induced fragmentations of this compound, providing evidence for an “ortho attack” in the molecular ion [].
Q3: What is the primary application of this compound in the chemical industry?
A3: this compound is a valuable intermediate in the synthesis of various fine chemicals and pharmaceuticals [].
Q4: Which reaction is commonly used to synthesize this compound?
A4: this compound is primarily synthesized through the selective hydrogenation of cinnamaldehyde [, , , , , , , , , , , , , , , ].
Q5: What are the challenges associated with the selective hydrogenation of cinnamaldehyde to this compound?
A5: The selective hydrogenation of cinnamaldehyde is challenging because both the C=C and C=O bonds can be reduced. Achieving high selectivity towards this compound (C=C hydrogenation) requires careful control of reaction conditions and catalyst design [, , , , , , ].
Q6: What types of catalysts are used for the selective hydrogenation of cinnamaldehyde to this compound?
A6: A variety of catalysts have been explored, including:
- Palladium-based catalysts: These are widely studied due to their high activity and selectivity for C=C hydrogenation. Researchers have investigated the effect of support materials like activated carbon, SiO2, TiO2, γ-Al2O3, SiC, and graphene oxide on the performance of Pd catalysts [, , , , , , ]. Bimetallic Pd catalysts, such as Pd-Ni [] and Pd-Ag [], have also been studied to further improve activity and selectivity.
- Nickel-based catalysts: Ni catalysts offer a more cost-effective alternative to Pd catalysts. Supported Ni catalysts, such as Ni/SiO2 [] and Ni/La2O3 [], have been reported for this compound production.
- Other catalysts: Copper-based catalysts, including Cu/SiO2 [] and bimetallic Ag-Ru/γ-Al2O3 [], have also demonstrated potential for selective cinnamaldehyde hydrogenation to this compound.
Q7: How does the choice of catalyst and reaction conditions affect the selectivity of cinnamaldehyde hydrogenation?
A7:
- Catalyst: The size of Pd nanoparticles significantly influences selectivity. Smaller Pd particles favor C=C hydrogenation to this compound, while larger particles lead to a mixture of this compound, cinnamyl alcohol (C=O hydrogenation), and 3-phenylpropanol (full hydrogenation) [, ]. The presence of a second metal in bimetallic catalysts can also enhance selectivity by modifying the electronic properties and adsorption characteristics of the active sites [, , ].
- Support material: The support material can impact the dispersion and electronic properties of the metal nanoparticles, influencing catalyst activity and selectivity [, , , , , ]. For instance, strong metal-support interactions (SMSI) in Pd/TiO2 catalysts can affect Pd particle size and consequently alter selectivity [].
- Reaction conditions: Factors like temperature, pressure, solvent, and the presence of additives can significantly influence the selectivity of the reaction [, , , , ]. For instance, supercritical carbon dioxide as a solvent has shown remarkable enhancement in selectivity towards cinnamyl alcohol over a Ru-Pt bimetallic catalyst compared to conventional solvents [].
Q8: What alternative synthetic routes exist for this compound production?
A8: While less common, alternative methods for this compound synthesis include:
- Reduction of cinnamaldehyde using specific reagents: Researchers have explored using cobalt carbonyl in the presence of amines to achieve high selectivity towards this compound [].
- Oxidation of 3-phenylpropanol: Lead tetraacetate oxidation of 3-phenylpropanol has been reported as a route to this compound [].
Q9: How does the use of a mechanochemically synthesized Pd/Al-SBA-15 catalyst affect the hydroconversion of cinnamaldehyde?
A9: Studies using mechanochemically synthesized Pd/Al-SBA-15 catalysts revealed the presence of competitive pathways during cinnamaldehyde hydroconversion. These pathways can shift selectivity from fully hydrogenated products like cyclohexane and this compound to unexpected products like ethylbenzene and oxalic acid, resulting from hydrodeformylation and hydrocarboxylation reactions, respectively [].
Q10: Is there information available about the compatibility of this compound with different materials?
A10: The provided research papers primarily focus on the synthesis and catalytic applications of this compound. While specific information about material compatibility isn't directly discussed, it's important to note that aldehydes can react with various functional groups, potentially limiting compatibility with certain materials. Further research might be needed to ascertain the compatibility of this compound with specific materials under diverse conditions.
Q11: What is known about the stability of this compound under various conditions?
A11: While the provided papers don't delve into the detailed stability profile of this compound, it's generally understood that aldehydes can undergo oxidation, polymerization, and aldol condensation reactions under certain conditions. Factors like temperature, exposure to air and light, and the presence of acids or bases can influence its stability. Further research might be needed to establish specific stability data for this compound under different conditions.
Q12: Have there been any computational studies on the hydrogenation of cinnamaldehyde?
A12: Yes, Density Functional Theory (DFT) calculations have been employed to understand the adsorption behavior of cinnamaldehyde on palladium surfaces. These calculations help explain the observed selectivity differences with varying Pd particle sizes [].
Q13: How do structural modifications of cinnamaldehyde influence the selectivity of its hydrogenation?
A13: Structural modifications, particularly those influencing the steric environment around the C=C and C=O bonds, can impact the adsorption mode of the molecule on the catalyst surface, thereby influencing selectivity []. For instance, increasing the steric bulk around the C=O group might hinder its adsorption, favoring C=C hydrogenation and leading to higher this compound selectivity.
Q14: Is there information on how the structure of this compound relates to its properties or applications?
A14: The research papers focus on the synthesis of this compound and its use as an intermediate in the production of other compounds. Detailed structure-activity relationship studies focusing on this compound itself are not presented in these papers.
Q15: What analytical techniques are commonly used to monitor the hydrogenation of cinnamaldehyde and quantify this compound?
A15: Researchers primarily rely on gas chromatography (GC) coupled with various detectors, such as a mass selective detector (MSD) or a flame ionization detector (FID), to monitor the reaction progress and quantify this compound alongside other products [, , , ].
Q16: Is there information on the environmental impact and degradation of this compound?
A16: The provided research papers primarily focus on the chemical aspects and applications of this compound. Data regarding its environmental impact, degradation pathways, and potential ecotoxicological effects are not extensively discussed. Further investigation is required to assess its environmental fate and potential risks.
Q17: Are there any known alternatives to this compound for its specific applications?
A17: The research papers don't explicitly discuss alternatives to this compound. The choice of alternative compounds would depend on the specific application and the desired properties.
Q18: What research tools and resources have been essential for advancing our understanding of this compound?
A18: Key research tools and resources include:
- Catalyst synthesis and characterization: Techniques like impregnation, solvothermal synthesis, and mechanochemical synthesis have been crucial for preparing diverse catalysts. Characterization techniques like XRD, TEM, XPS, TPR, and FTIR spectroscopy provide valuable insights into catalyst structure, composition, and active sites [, , , , , , , , , , ].
- Reaction monitoring and product analysis: Gas chromatography (GC) equipped with various detectors (MSD, FID) enables the identification and quantification of reaction products, facilitating kinetic studies and selectivity assessments [, , , ].
- Computational chemistry: Density functional theory (DFT) calculations provide insights into molecular adsorption behavior on catalyst surfaces, aiding in the understanding of reaction mechanisms and selectivity trends [].
Disclaimer and Information on In-Vitro Research Products
Please be aware that all articles and product information presented on BenchChem are intended solely for informational purposes. The products available for purchase on BenchChem are specifically designed for in-vitro studies, which are conducted outside of living organisms. In-vitro studies, derived from the Latin term "in glass," involve experiments performed in controlled laboratory settings using cells or tissues. It is important to note that these products are not categorized as medicines or drugs, and they have not received approval from the FDA for the prevention, treatment, or cure of any medical condition, ailment, or disease. We must emphasize that any form of bodily introduction of these products into humans or animals is strictly prohibited by law. It is essential to adhere to these guidelines to ensure compliance with legal and ethical standards in research and experimentation.