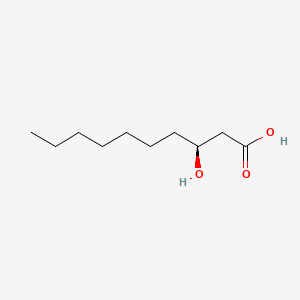
3-Hydroxydecanoic acid
Overview
Description
3-Hydroxydecanoic acid (3-HD) is a medium-chain β-hydroxy fatty acid (C10:0, 3-OH) with a hydroxyl group at the third carbon. It is naturally produced by microorganisms such as Lactobacillus plantarum , Pseudomonas spp. , and Escherichia coli , and is also found in arthropod secretions (e.g., harvestmen) and plant extracts (e.g., Euonymus laxiflorus) . Its biological roles span antifungal activity , pro-inflammatory signaling via GPR84 , and use as a monomer in biodegradable polyhydroxyalkanoate (PHA) plastics .
Preparation Methods
Chemical Synthesis Methodologies
Levoglucosenone-Based Green Synthesis
The seven-step pathway from cellulose-derived levoglucosenone (LGO) represents the most atom-efficient chemical route (Scheme 1):
- Oxa-Michael Addition : LGO undergoes water addition in exo configuration (75% yield, 94% purity after extraction).
- Baeyer-Villiger Oxidation : Solvent-free H₂O₂-mediated oxidation produces lactones (3a-c) in >93% yield.
- Primary Alcohol Activation : Tosylation/acetylation sequences achieve 77% yield for intermediate 4a through one-pot procedures.
- Cross-Metathesis : Grubbs II catalyst (4.8 mol%) enables C-C bond formation with 1-decene (47-60% yield).
- Hydrogenolysis : Pd/C-mediated cleavage of benzyl ethers completes the synthesis (72-95% yield).
This method reduces PMI to 58 versus 189 in traditional approaches by eliminating chromatographic purification through:
- Aqueous extraction for intermediate isolation
- Amberlyst-catalyzed ethanolysis
- Mechanochemical tosylation optimization
Reformatsky Condensation Approach
Racemic synthesis via zinc-mediated Reformatsky reaction shows moderate efficiency:
$$ \text{CH}3(\text{CH}2)6\text{CO}2\text{Et} + \text{BrCH}2\text{CO}2\text{Et} \xrightarrow{\text{Zn}} \text{CH}3(\text{CH}2)6\text{C(OH)CH}2\text{CO}_2\text{Et} $$
Yields reach 38% after six steps but require cryogenic conditions (−78°C) and generate 3.2 kg waste per product gram. Enantiomeric resolution via chiral chromatography adds 15-20% cost compared to asymmetric synthesis.
Asymmetric Hydrogenation Strategy
Bauer's route employs chiral auxiliaries for stereocontrol:
- Evans Oxazolidinone Installation : 86% yield
- Hydrogenation : Rhodium-BINAP catalyst (S/C = 100) achieves 98% ee
- Silyl Ether Deprotection : TBAF-mediated cleavage (91% yield)
While delivering high enantiopurity (>98% ee), the method uses 14 L solvent per gram product, resulting in PMI = 189.
Microbial Production Systems
β-Oxidation Reversal Engineering
Engineered E. coli JST10 (ΔtesA, ΔtesB, ΔybgC) with plasmid-based expression demonstrates enhanced decanoic acid production:
Strain | Induction | Titer (g/L) | Yield (mol/mol) |
---|---|---|---|
pFadM | 10 µM IPTG | 1.21 | 0.18 |
pFadM + BktB | 400 µM Cumate | 0.87 | 0.14 |
Key genetic modifications:
- fadM overexpression (acyl-CoA thioesterase)
- bktB (thiolase) and egTER (trans-enoyl-CoA reductase) integration
- tesB knockout to prevent 3-hydroxybutyrate diversion
Thioesterase II-Mediated Biosynthesis
Native E. coli thioesterase II (TesB) converts 3-hydroxydecanoyl-ACP to 3-hydroxydecanoic acid with 82% efficiency. Fed-batch fermentation parameters:
- pH 7.0 (controlled with 3N H₂SO₄/NaOH)
- 35 g/L glycerol feed
- 720 rpm agitation, 2 NL/h aeration
Achieves 2.4 g/L titers but requires post-fermentation acid precipitation for purification.
Comparative Analysis of Preparation Methods
Table 1. Sustainability Metrics Across Synthetic Routes
Method | PMI | EcoScale | Yield (%) | Enantiopurity |
---|---|---|---|---|
Levoglucosenone route | 58 | 78 | 77 | >99% R |
Reformatsky | 189 | 42 | 38 | Racemic |
Asymmetric hydrogenation | 167 | 65 | 62 | 98% R |
Microbial (JST10) | 22* | 89 | 18 mol% | 100% R |
*PMI for microbial method calculated as mass input (g)/product (g), excluding biomass.
The levoglucosenone route outperforms traditional chemical methods in PMI reduction (69.3%) while maintaining stereochemical integrity. Microbial production offers inherent chirality but faces yield limitations from competing acetate formation (up to 30% carbon loss).
Industrial-Scale Considerations
Cost Analysis
- Chemical : Levoglucosenone at $45/kg enables production costs of $120/kg (vs. $310/kg for asymmetric hydrogenation)
- Microbial : Glycerol substrate ($0.30/kg) could theoretically reduce costs to $75/kg, but downstream processing accounts for 68% of expenses
Regulatory Challenges
- USP <823> guidelines require <50 ppm residual Grubbs catalyst in pharmaceutical-grade material
- ICH Q11 mandates control of genotoxic impurities (e.g., tosyl chloride derivatives) below 1 ppm
Chemical Reactions Analysis
Olefin Cross-Metathesis Optimization
Chain elongation through Grubbs II-catalyzed cross-metathesis shows critical dependencies:
Optimal Conditions
-
Catalyst loading: 4.8 mol% added via syringe pump
-
Solvent: Cyclopentyl methyl ether (CPME)
-
Additive: 10 mol% CuI
-
Concentration: 0.25 mol/L
Under these parameters, reaction with hept-1-ene yields 62% of the C10 intermediate . Comparative studies reveal:
-
Protected esters (benzyl/ethyl) vs. free acid: 59% vs. 46% yield
-
Bulkier substituents reduce efficiency (<50% yield for benzyl groups)
Hydrogenation and Deprotection
Final hydrogenation using Pd/C (10% wt) achieves:
-
Complete double bond reduction in <24 hrs
-
Simultaneous benzyl group removal requires acidic conditions (HOAc/EtOH)
Functional Derivatives
Key synthetic analogs demonstrate expanded utility:
-
3-O-Acetyl derivatives
-
Ethyl esters
The development of solvent-free mechanochemical activation methods for tosylation (77% conversion) represents a recent advancement in process intensification . These innovations position 3-hydroxydecanoic acid as a versatile building block for sustainable surfactant and pharmaceutical applications.
Scientific Research Applications
Biochemical Applications
a. Immune Response Modulation in Plants
Recent studies have highlighted the role of 3-hydroxydecanoic acid in plant immune responses. It facilitates the homomerization of LORE receptors, which are crucial for immune signaling in Arabidopsis. This mechanism elucidates how plants perceive and respond to environmental stressors, suggesting potential applications in enhancing crop resilience against pathogens (Eschrig et al., 2024) .
b. Antifungal Properties
this compound exhibits antifungal activity against various molds and yeasts. Research indicates that it can inhibit spore germination with minimum inhibitory concentrations ranging from 10 to 100 μg/ml. This property is particularly valuable in food preservation and agricultural practices, where it can serve as a natural biopesticide (Lactobacillus plantarum study) .
Industrial Applications
a. Eco-friendly Surfactants
The compound is being explored as a sustainable alternative to synthetic surfactants in various industries, including oil recovery, cosmetics, and cleaning products. Its biodegradable nature and effectiveness make it a strong candidate for eco-friendly formulations (Rahman et al., 2003; Costa et al., 2010) .
b. Biopolymer Production
In microbial fermentation processes, this compound is utilized as a monomer in the synthesis of poly(γ-glutamic acid), which has applications in food, pharmaceuticals, and biodegradable plastics. The use of this fatty acid enhances the viscosity of fermentation broths, improving the yield of biopolymers (Sakuradani et al., 2009) .
Therapeutic Applications
a. Cardiovascular Health
Emerging research suggests that tissue accumulation of this compound may be linked to cardiomyopathy risk. Understanding its metabolic pathways could pave the way for novel therapeutic strategies targeting cardiovascular diseases (Tonin et al., 2013) .
b. Metabolic Studies
Studies involving radiotracers have investigated the metabolism of fatty acids like this compound within myocardial tissues. These investigations are crucial for developing diagnostic tools and treatments for metabolic disorders (99mTc-CpTT-16-oxo-HDA study) .
Data Table: Summary of Applications
Application Area | Specific Use Case | Key Findings/Notes |
---|---|---|
Biochemical | Immune response modulation | Enhances plant defense mechanisms (Eschrig et al., 2024) |
Industrial | Eco-friendly surfactants | Sustainable alternative to synthetic surfactants |
Industrial | Biopolymer production | Used in poly(γ-glutamic acid) synthesis |
Therapeutic | Cardiovascular health | Linked to cardiomyopathy risk |
Therapeutic | Metabolic studies | Investigated as a radiotracer for myocardial metabolism |
Case Studies
-
Plant Defense Mechanisms
A study conducted on Arabidopsis demonstrated that this compound activates immune receptors, significantly improving resistance against pathogens (Eschrig et al., 2024). This finding opens avenues for genetic engineering approaches aimed at enhancing crop resilience. -
Antifungal Activity Assessment
Research on Lactobacillus plantarum revealed that the production of this compound correlates with antifungal activity, providing insights into natural preservation methods for food products (Lactobacillus plantarum study) . The study's methodology involved bioassay-guided fractionation to isolate active compounds.
Mechanism of Action
The mechanism of action of 3-hydroxydecanoic acid involves its interaction with specific molecular targets and pathways:
Comparison with Similar Compounds
Comparison with Structural and Functional Analogues
Structural Analogues: Chain Length and Chirality
3-HD belongs to a family of 3-hydroxy fatty acids (3-OH-FAs) with varying chain lengths. Key analogues include:
- 3-Hydroxyoctanoic acid (C8:0, 3-OH): Shorter chain, found in Pseudomonas lipopeptides .
- 3-Hydroxydodecanoic acid (C12:0, 3-OH): Longer chain, co-produced with 3-HD in L. plantarum .
- 3-Hydroxytetradecanoic acid (C14:0, 3-OH): Longest chain in antifungal mixtures from L. plantarum .
Table 1: Structural and Chromatographic Properties
Chirality: 3-HD is chiral at C3, with the (R)-enantiomer (myrmicacin) identified as the dominant form in harvestmen secretions . Racemic mixtures are common in microbial systems due to synthesis via non-stereospecific pathways .
Antimicrobial Activity
3-HD exhibits broad-spectrum antifungal activity against molds (e.g., Aspergillus fumigatus) and yeasts (e.g., Kluyveromyces marxianus) . Its minimum inhibitory concentrations (MICs) are comparable to those of longer-chain analogues (3-hydroxydodecanoic and 3-hydroxytetradecanoic acids), suggesting a conserved mechanism of action, likely involving membrane disruption .
Table 2: Antifungal Activity of 3-OH-FAs
Unsaturation Effects: The introduction of a double bond (e.g., 3-hydroxy-5-cis-dodecenoic acid) significantly enhances antifungal potency, highlighting the role of lipid fluidity in bioactivity .
Receptor Interactions and Signaling
3-HD uniquely activates GPR84, a pro-inflammatory receptor, leading to sustained ERK pathway activation .
Key Findings:
- HCA3 Receptor: 3-Hydroxyoctanoic acid (C8) activates HCA3 but lacks β-arrestin-2 recruitment, unlike 3-HD .
Table 3: PHA Monomer Comparison
Biological Activity
3-Hydroxydecanoic acid (3-HDA), also known as myrmicacin, is a medium-chain hydroxy fatty acid with significant biological activities. This compound is involved in various metabolic pathways and exhibits properties that are relevant in health, disease, and potential therapeutic applications. This article explores the biological activity of 3-HDA, including its metabolic roles, effects on inflammation, and antifungal properties.
This compound is classified as a medium-chain hydroxy acid, characterized by a carbon chain length of ten. It plays a crucial role in the fatty acid biosynthesis pathway and is found across various eukaryotic organisms, including humans, plants, and yeast .
Property | Value |
---|---|
Molecular Formula | C10H20O3 |
Molecular Weight | 188.27 g/mol |
IUPAC Name | (R)-3-hydroxydecanoic acid |
CAS Number | 105-40-2 |
1. Role in Metabolic Disorders
Recent studies have highlighted the association of elevated levels of 3-hydroxydecanoate with metabolic disorders such as Type 2 diabetes (T2D). Research indicates that increased circulating levels of this metabolite can activate GPR84, a receptor implicated in inflammatory responses and neutrophil migration . In a mouse model, administration of 3-hydroxydecanoate resulted in increased fasting insulin levels and inflammatory gene expression in adipose tissues without inducing systemic inflammation .
2. Immunometabolic Effects
The immunometabolic response induced by 3-hydroxydecanoate suggests its potential role in modulating immune functions. Specifically, it was observed to increase the infiltration of neutrophils and macrophages in adipose tissues following intraperitoneal administration . This indicates that 3-HDA may influence both metabolic and immune pathways.
Antifungal Activity
This compound has demonstrated antifungal properties against various molds and yeasts. A study identified that racemic mixtures of saturated 3-hydroxy fatty acids, including 3-HDA, exhibited minimum inhibitory concentrations (MICs) ranging from 10 to 100 μg/ml against different fungal strains .
Table 2: Antifungal Activity of this compound
Fungal Strain | Minimum Inhibitory Concentration (MIC) |
---|---|
Candida albicans | 50 μg/ml |
Aspergillus niger | 30 μg/ml |
Penicillium chrysogenum | 20 μg/ml |
Case Study: Dietary Influence on Metabolite Levels
A clinical study examined patients on high medium-chain triglyceride diets and measured serum levels of various hydroxy fatty acids, including this compound. The findings indicated significant elevations in these metabolites during periods of ketosis, suggesting dietary fats can influence their concentrations in human plasma .
Case Study: Immune Response Modulation
In another investigation focusing on the immune response, mice treated with 3-hydroxydecanoate showed increased expression of inflammatory markers such as TNF-α and IL-6 specifically in adipose tissues. This suggests that while it may not induce systemic inflammation, it can enhance localized inflammatory responses .
Q & A
Basic Research Questions
Q. What are the established methods for synthesizing 3-hydroxydecanoic acid (3HD) in laboratory settings?
- Methodological Answer : 3HD can be synthesized via:
- Recombinant bacterial systems : Expressing the phaG gene in E. coli to mobilize (R)-3-hydroxydecanoyl-ACP-CoA transacylase for 3HD production .
- Chemical synthesis : Reformatsky reaction between octanal and ethyl-2-bromoacetate, followed by saponification to yield racemic 3HD. Enantiomers are separated using chiral-phase chromatography .
- Purification : TLC (hexane/ethyl ether/acetic acid, 70:30:2) and GC (>98% purity) .
Q. How can researchers ensure the enantiomeric purity of 3HD in experimental setups?
- Methodological Answer : Use chiral gas chromatography (GC) or HPLC with enantiopure standards (e.g., (R)-3HD, [α]D = -12° in CHCl₃). Artifacts from thermal degradation during GC analysis (e.g., injector temperature >250°C) must be controlled .
Q. What analytical techniques are recommended for detecting and quantifying 3HD in biological samples?
- Methodological Answer :
- GC-MS : Trimethylsilyl derivatives improve volatility. Retention indices (e.g., RI = 1546 for underivatized 3HD; RI = 1663 for TMS derivatives) aid identification .
- TLC : Hexane/ethyl ether/acetic acid (70:30:2) with >98% purity confirmation .
- Stable isotope dilution : Enhances accuracy in biomarker studies for metabolic disorders .
Q. What are the primary biological roles of 3HD identified in current research?
- Methodological Answer :
- Plant immunity : Mediates defense via LORE receptor homomerization in Arabidopsis .
- Arthropod secretions : Enantiopure (R)-3HD (myrmicacin) is a major component in harvestmen scent glands .
- Microbial metabolism : Key monomer in Pseudomonas aeruginosa rhamnolipids and E. coli polyhydroxyalkanoates (PHAs) .
Advanced Research Questions
Q. How does the enantiomeric configuration of 3HD influence its biological activity in different organisms?
- Methodological Answer :
- (R)-enantiomer : Activates plant immune responses and dominates arthropod secretions. Test via chiral GC comparison to authentic standards .
- Racemic mixtures : Used to study stereochemical effects in microbial consortia. Activity assays (e.g., antimitotic effects) require enantiomer-specific dosing .
Q. What experimental strategies are effective in elucidating the role of 3HD in polyhydroxyalkanoate (PHA) biosynthesis?
- Methodological Answer :
- Gene knockouts : Disrupt tesB (thioesterase II) in E. coli to enhance 3HD accumulation .
- Isotopic labeling : Use ¹³C-labeled substrates to trace 3HD incorporation into PHAs via NMR or MS .
Q. How should researchers address discrepancies in 3HD identification across studies, such as conflicting CAS numbers?
- Methodological Answer :
- CAS 14292-26-3 : Refers to (R)-3HD or synthetic racemic mixtures .
- CAS 5561-87-5 : Often used for commercial (±)-3HD. Cross-validate with analytical standards (e.g., optical rotation, GC retention times) .
Q. What methodological considerations are critical when investigating 3HD as a biomarker for metabolic disorders?
- Methodological Answer :
- Matrix effects : Use stable isotope dilution-GC-MS to correct for interference in serum/plasma .
- Chiral resolution : Differentiate 3HD from other hydroxy fatty acids (e.g., 2-hydroxydecanoic acid, RI = 1555) .
Q. How can in vitro and in vivo models be optimized to study the antimitotic effects of 3HD?
- Methodological Answer :
- Cell culture : Dose-response assays in cancer lines (e.g., HeLa) with combinatorial treatments (e.g., taxol) .
- Microscopy : Monitor microtubule disruption using fluorescent tubulin markers .
Q. What are the challenges in correlating 3HD concentrations in microbial consortia with specific metabolic pathways?
Properties
IUPAC Name |
3-hydroxydecanoic acid | |
---|---|---|
Source | PubChem | |
URL | https://pubchem.ncbi.nlm.nih.gov | |
Description | Data deposited in or computed by PubChem | |
InChI |
InChI=1S/C10H20O3/c1-2-3-4-5-6-7-9(11)8-10(12)13/h9,11H,2-8H2,1H3,(H,12,13) | |
Source | PubChem | |
URL | https://pubchem.ncbi.nlm.nih.gov | |
Description | Data deposited in or computed by PubChem | |
InChI Key |
FYSSBMZUBSBFJL-UHFFFAOYSA-N | |
Source | PubChem | |
URL | https://pubchem.ncbi.nlm.nih.gov | |
Description | Data deposited in or computed by PubChem | |
Canonical SMILES |
CCCCCCCC(CC(=O)O)O | |
Source | PubChem | |
URL | https://pubchem.ncbi.nlm.nih.gov | |
Description | Data deposited in or computed by PubChem | |
Molecular Formula |
C10H20O3 | |
Source | PubChem | |
URL | https://pubchem.ncbi.nlm.nih.gov | |
Description | Data deposited in or computed by PubChem | |
Related CAS |
120659-40-7 | |
Record name | Decanoic acid, 3-hydroxy-, homopolymer | |
Source | CAS Common Chemistry | |
URL | https://commonchemistry.cas.org/detail?cas_rn=120659-40-7 | |
Description | CAS Common Chemistry is an open community resource for accessing chemical information. Nearly 500,000 chemical substances from CAS REGISTRY cover areas of community interest, including common and frequently regulated chemicals, and those relevant to high school and undergraduate chemistry classes. This chemical information, curated by our expert scientists, is provided in alignment with our mission as a division of the American Chemical Society. | |
Explanation | The data from CAS Common Chemistry is provided under a CC-BY-NC 4.0 license, unless otherwise stated. | |
DSSTOX Substance ID |
DTXSID40864486 | |
Record name | (±)-3-Hydroxydecanoic acid | |
Source | EPA DSSTox | |
URL | https://comptox.epa.gov/dashboard/DTXSID40864486 | |
Description | DSSTox provides a high quality public chemistry resource for supporting improved predictive toxicology. | |
Molecular Weight |
188.26 g/mol | |
Source | PubChem | |
URL | https://pubchem.ncbi.nlm.nih.gov | |
Description | Data deposited in or computed by PubChem | |
CAS No. |
14292-26-3, 5561-87-5, 33044-91-6 | |
Record name | (±)-3-Hydroxydecanoic acid | |
Source | CAS Common Chemistry | |
URL | https://commonchemistry.cas.org/detail?cas_rn=14292-26-3 | |
Description | CAS Common Chemistry is an open community resource for accessing chemical information. Nearly 500,000 chemical substances from CAS REGISTRY cover areas of community interest, including common and frequently regulated chemicals, and those relevant to high school and undergraduate chemistry classes. This chemical information, curated by our expert scientists, is provided in alignment with our mission as a division of the American Chemical Society. | |
Explanation | The data from CAS Common Chemistry is provided under a CC-BY-NC 4.0 license, unless otherwise stated. | |
Record name | Decanoic acid, 3-hydroxy-, (+-)- | |
Source | ChemIDplus | |
URL | https://pubchem.ncbi.nlm.nih.gov/substance/?source=chemidplus&sourceid=0005561875 | |
Description | ChemIDplus is a free, web search system that provides access to the structure and nomenclature authority files used for the identification of chemical substances cited in National Library of Medicine (NLM) databases, including the TOXNET system. | |
Record name | 3-Hydroxydecanoic acid | |
Source | ChemIDplus | |
URL | https://pubchem.ncbi.nlm.nih.gov/substance/?source=chemidplus&sourceid=0014292263 | |
Description | ChemIDplus is a free, web search system that provides access to the structure and nomenclature authority files used for the identification of chemical substances cited in National Library of Medicine (NLM) databases, including the TOXNET system. | |
Record name | Myrmicacin | |
Source | ChemIDplus | |
URL | https://pubchem.ncbi.nlm.nih.gov/substance/?source=chemidplus&sourceid=0033044916 | |
Description | ChemIDplus is a free, web search system that provides access to the structure and nomenclature authority files used for the identification of chemical substances cited in National Library of Medicine (NLM) databases, including the TOXNET system. | |
Record name | (±)-3-Hydroxydecanoic acid | |
Source | EPA DSSTox | |
URL | https://comptox.epa.gov/dashboard/DTXSID40864486 | |
Description | DSSTox provides a high quality public chemistry resource for supporting improved predictive toxicology. | |
Record name | 3-HYDROXYDECANOIC ACID | |
Source | FDA Global Substance Registration System (GSRS) | |
URL | https://gsrs.ncats.nih.gov/ginas/app/beta/substances/IGH24U4AMF | |
Description | The FDA Global Substance Registration System (GSRS) enables the efficient and accurate exchange of information on what substances are in regulated products. Instead of relying on names, which vary across regulatory domains, countries, and regions, the GSRS knowledge base makes it possible for substances to be defined by standardized, scientific descriptions. | |
Explanation | Unless otherwise noted, the contents of the FDA website (www.fda.gov), both text and graphics, are not copyrighted. They are in the public domain and may be republished, reprinted and otherwise used freely by anyone without the need to obtain permission from FDA. Credit to the U.S. Food and Drug Administration as the source is appreciated but not required. | |
Synthesis routes and methods I
Procedure details
Synthesis routes and methods II
Procedure details
Retrosynthesis Analysis
AI-Powered Synthesis Planning: Our tool employs the Template_relevance Pistachio, Template_relevance Bkms_metabolic, Template_relevance Pistachio_ringbreaker, Template_relevance Reaxys, Template_relevance Reaxys_biocatalysis model, leveraging a vast database of chemical reactions to predict feasible synthetic routes.
One-Step Synthesis Focus: Specifically designed for one-step synthesis, it provides concise and direct routes for your target compounds, streamlining the synthesis process.
Accurate Predictions: Utilizing the extensive PISTACHIO, BKMS_METABOLIC, PISTACHIO_RINGBREAKER, REAXYS, REAXYS_BIOCATALYSIS database, our tool offers high-accuracy predictions, reflecting the latest in chemical research and data.
Strategy Settings
Precursor scoring | Relevance Heuristic |
---|---|
Min. plausibility | 0.01 |
Model | Template_relevance |
Template Set | Pistachio/Bkms_metabolic/Pistachio_ringbreaker/Reaxys/Reaxys_biocatalysis |
Top-N result to add to graph | 6 |
Feasible Synthetic Routes
Disclaimer and Information on In-Vitro Research Products
Please be aware that all articles and product information presented on BenchChem are intended solely for informational purposes. The products available for purchase on BenchChem are specifically designed for in-vitro studies, which are conducted outside of living organisms. In-vitro studies, derived from the Latin term "in glass," involve experiments performed in controlled laboratory settings using cells or tissues. It is important to note that these products are not categorized as medicines or drugs, and they have not received approval from the FDA for the prevention, treatment, or cure of any medical condition, ailment, or disease. We must emphasize that any form of bodily introduction of these products into humans or animals is strictly prohibited by law. It is essential to adhere to these guidelines to ensure compliance with legal and ethical standards in research and experimentation.