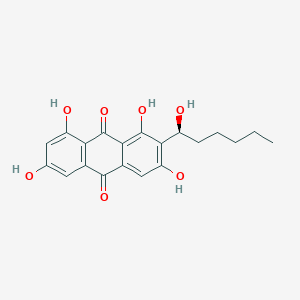
Averantin
Overview
Description
Averantin is a polyketide compound produced by certain species of the fungus Aspergillus, particularly Aspergillus parasiticus and Aspergillus flavus. It is an intermediate in the biosynthetic pathway of aflatoxins, which are highly toxic and carcinogenic secondary metabolites. This compound plays a crucial role in the conversion of norsolorinic acid to aflatoxins, making it an important compound in the study of fungal metabolism and toxin production .
Mechanism of Action
Target of Action
Averantin, also known as (-)-Averantin, is a secondary metabolite produced by certain fungi, including Aspergillus species . It serves as a precursor in the biosynthesis of aflatoxin B1 , a potent mycotoxin. The primary target of this compound is the aflatoxin biosynthetic pathway .
Mode of Action
The mode of action of this compound involves its conversion into other compounds in the aflatoxin biosynthetic pathway . The nor1
gene encodes for ketoreductase that reduces the primary keto group of the NOR hydroxyl group and leads to the production of this compound . Other genes that can be used for this reduction reaction are homologous norA
and norB
, which encode for short-chain aryl alcohol dehydrogenase .
Biochemical Pathways
This compound is involved in the aflatoxin biosynthetic pathway . It is converted from norsolorinic acid, a step governed by the aflD
gene . This compound is then further transformed into other compounds, including averufin . This complex pathway involves at least 27 enzymatic reactions .
Result of Action
The result of this compound’s action is the production of aflatoxin B1, a potent mycotoxin . Aflatoxin B1 is highly toxic and carcinogenic, posing significant health risks when ingested by humans or animals .
Action Environment
The production of this compound and its conversion into aflatoxin B1 can be influenced by various environmental factors . These include nutritional factors such as carbon and nitrogen source, environmental effects such as water activity and temperature, and physiological conditions such as pH . Understanding these factors can help in developing strategies to control aflatoxin contamination.
Biochemical Analysis
Biochemical Properties
Averantin plays a crucial role in the biosynthesis of aflatoxin . It is converted to averufin in aflatoxin biosynthesis, a process catalyzed by the enzyme cytochrome P450 monooxygenase . This interaction involves the reduction of the keto group of norsolorinic acid to form this compound .
Cellular Effects
This compound and its derivative, 1’-O-methyl-averantin, have shown cytotoxic activity against various human cancer cell lines . They have been found to suppress spheroid formation in colorectal cancer cell lines and downregulate the expression of stemness markers .
Molecular Mechanism
The molecular mechanism of this compound involves its conversion to 5’-hydroxy-averantin by the enzyme AflG, a cytochrome P450 monooxygenase . This conversion is a crucial step in aflatoxin biosynthesis .
Metabolic Pathways
This compound is involved in the metabolic pathway of aflatoxin biosynthesis . It is converted from norsolorinic acid by a cytosol oxidoreductase in the presence of NADPH or NADH .
Transport and Distribution
As a precursor in the aflatoxin biosynthesis pathway, it is likely to be transported and distributed within fungal cells where this pathway occurs .
Subcellular Localization
The enzymes involved in its conversion, such as the cytochrome P450 monooxygenase AflG, are likely to be located in the cytosol .
Preparation Methods
Synthetic Routes and Reaction Conditions
The synthesis of averantin involves the reduction of norsolorinic acid by a cytosol oxidoreductase enzyme in the presence of NADPH or NADH. This reaction is reversible when NADP or NAD is present . The process is typically carried out under controlled laboratory conditions to ensure the proper conversion of norsolorinic acid to this compound.
Industrial Production Methods
Industrial production of this compound is not common due to its role as an intermediate in the biosynthesis of aflatoxins. it can be produced in large quantities through the cultivation of Aspergillus species under specific conditions that promote the production of aflatoxin intermediates. This involves maintaining optimal temperature, humidity, and nutrient availability to support fungal growth and metabolite production .
Chemical Reactions Analysis
Types of Reactions
Averantin undergoes several types of chemical reactions, including:
Oxidation: This compound can be oxidized to form 5’-hydroxythis compound, which is then further converted to averufin.
Reduction: The reduction of norsolorinic acid to this compound is a key step in its biosynthesis.
Substitution: Various substitution reactions can occur on the anthraquinone core of this compound, leading to the formation of different derivatives.
Common Reagents and Conditions
Oxidation: P-450 monooxygenase enzymes are commonly used for the oxidation of this compound to 5’-hydroxythis compound.
Reduction: NADPH or NADH are essential cofactors for the reduction of norsolorinic acid to this compound.
Major Products Formed
5’-Hydroxythis compound: Formed through the oxidation of this compound.
Averufin: Produced from the further conversion of 5’-hydroxythis compound.
Scientific Research Applications
Averantin is primarily studied in the context of aflatoxin biosynthesis. Its role as an intermediate makes it a valuable compound for understanding the enzymatic pathways and genetic regulation involved in toxin production. Research on this compound contributes to the development of strategies to control aflatoxin contamination in food and feed, which is a significant concern for food safety and public health .
The unique chemical structure of this compound allows for the exploration of its biological activities, including antimicrobial and anticancer properties .
Comparison with Similar Compounds
Averantin is part of a larger group of anthraquinone derivatives produced by Aspergillus species. Similar compounds include:
Norsolorinic Acid: The precursor to this compound in the aflatoxin biosynthetic pathway.
5’-Hydroxythis compound: An intermediate formed from the oxidation of this compound.
Averufin: A downstream product in the aflatoxin biosynthetic pathway.
Compared to these compounds, this compound is unique in its specific role as an intermediate that bridges the conversion of norsolorinic acid to more advanced aflatoxin precursors. Its study provides insights into the early stages of aflatoxin biosynthesis and the enzymatic mechanisms involved .
Properties
IUPAC Name |
1,3,6,8-tetrahydroxy-2-(1-hydroxyhexyl)anthracene-9,10-dione | |
---|---|---|
Details | Computed by Lexichem TK 2.7.0 (PubChem release 2021.05.07) | |
Source | PubChem | |
URL | https://pubchem.ncbi.nlm.nih.gov | |
Description | Data deposited in or computed by PubChem | |
InChI |
InChI=1S/C20H20O7/c1-2-3-4-5-12(22)17-14(24)8-11-16(20(17)27)19(26)15-10(18(11)25)6-9(21)7-13(15)23/h6-8,12,21-24,27H,2-5H2,1H3 | |
Details | Computed by InChI 1.0.6 (PubChem release 2021.05.07) | |
Source | PubChem | |
URL | https://pubchem.ncbi.nlm.nih.gov | |
Description | Data deposited in or computed by PubChem | |
InChI Key |
WGPOPPKSQRZUTP-UHFFFAOYSA-N | |
Details | Computed by InChI 1.0.6 (PubChem release 2021.05.07) | |
Source | PubChem | |
URL | https://pubchem.ncbi.nlm.nih.gov | |
Description | Data deposited in or computed by PubChem | |
Canonical SMILES |
CCCCCC(C1=C(C=C2C(=C1O)C(=O)C3=C(C2=O)C=C(C=C3O)O)O)O | |
Details | Computed by OEChem 2.3.0 (PubChem release 2021.05.07) | |
Source | PubChem | |
URL | https://pubchem.ncbi.nlm.nih.gov | |
Description | Data deposited in or computed by PubChem | |
Molecular Formula |
C20H20O7 | |
Details | Computed by PubChem 2.1 (PubChem release 2021.05.07) | |
Source | PubChem | |
URL | https://pubchem.ncbi.nlm.nih.gov | |
Description | Data deposited in or computed by PubChem | |
DSSTOX Substance ID |
DTXSID50973614 | |
Record name | 1,3,6,8-Tetrahydroxy-2-(1-hydroxyhexyl)anthracene-9,10-dione | |
Source | EPA DSSTox | |
URL | https://comptox.epa.gov/dashboard/DTXSID50973614 | |
Description | DSSTox provides a high quality public chemistry resource for supporting improved predictive toxicology. | |
Molecular Weight |
372.4 g/mol | |
Details | Computed by PubChem 2.1 (PubChem release 2021.05.07) | |
Source | PubChem | |
URL | https://pubchem.ncbi.nlm.nih.gov | |
Description | Data deposited in or computed by PubChem | |
CAS No. |
5803-62-3 | |
Record name | 1,3,6,8-Tetrahydroxy-2-(1-hydroxyhexyl)anthracene-9,10-dione | |
Source | EPA DSSTox | |
URL | https://comptox.epa.gov/dashboard/DTXSID50973614 | |
Description | DSSTox provides a high quality public chemistry resource for supporting improved predictive toxicology. | |
Retrosynthesis Analysis
AI-Powered Synthesis Planning: Our tool employs the Template_relevance Pistachio, Template_relevance Bkms_metabolic, Template_relevance Pistachio_ringbreaker, Template_relevance Reaxys, Template_relevance Reaxys_biocatalysis model, leveraging a vast database of chemical reactions to predict feasible synthetic routes.
One-Step Synthesis Focus: Specifically designed for one-step synthesis, it provides concise and direct routes for your target compounds, streamlining the synthesis process.
Accurate Predictions: Utilizing the extensive PISTACHIO, BKMS_METABOLIC, PISTACHIO_RINGBREAKER, REAXYS, REAXYS_BIOCATALYSIS database, our tool offers high-accuracy predictions, reflecting the latest in chemical research and data.
Strategy Settings
Precursor scoring | Relevance Heuristic |
---|---|
Min. plausibility | 0.01 |
Model | Template_relevance |
Template Set | Pistachio/Bkms_metabolic/Pistachio_ringbreaker/Reaxys/Reaxys_biocatalysis |
Top-N result to add to graph | 6 |
Feasible Synthetic Routes
Disclaimer and Information on In-Vitro Research Products
Please be aware that all articles and product information presented on BenchChem are intended solely for informational purposes. The products available for purchase on BenchChem are specifically designed for in-vitro studies, which are conducted outside of living organisms. In-vitro studies, derived from the Latin term "in glass," involve experiments performed in controlled laboratory settings using cells or tissues. It is important to note that these products are not categorized as medicines or drugs, and they have not received approval from the FDA for the prevention, treatment, or cure of any medical condition, ailment, or disease. We must emphasize that any form of bodily introduction of these products into humans or animals is strictly prohibited by law. It is essential to adhere to these guidelines to ensure compliance with legal and ethical standards in research and experimentation.