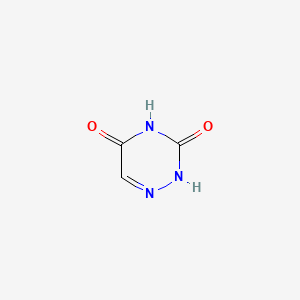
6-Azauracil
Overview
Description
6-Azauracil, also known as 1,2,4-triazine-3,5(2H,4H)-dione, is a heterocyclic compound that belongs to the class of triazines. It is structurally similar to uracil, a naturally occurring pyrimidine found in RNA. This compound has garnered significant interest due to its diverse biological activities, including antimicrobial, antiviral, and anticancer properties .
Mechanism of Action
Target of Action
6-Azauracil (6-AU) is a pyrimidine analog of uracil . It primarily targets enzymes involved in purine and pyrimidine biosynthesis . It competes with substrates in the pyrimidine biosynthetic pathway, being transformed into 6-aza-UMP, a powerful competitive inhibitor of orotidylic acid decarboxylase .
Mode of Action
6-AU inhibits the growth of various microorganisms by depleting intracellular guanosine triphosphate (GTP) and uridine triphosphate (UTP) nucleotide pools . It causes changes in the nucleoside pools, lowering GTP levels by inhibiting IMP dehydrogenase (Imd2), which participates in the first step of the GMP anabolic pathway, as well as in the UTP levels .
Biochemical Pathways
The biochemical pathways affected by 6-AU are primarily those involved in nucleotide biosynthesis. The compound competes with substrates in the pyrimidine biosynthetic pathway, leading to the production of 6-aza-UMP, which inhibits orotidylic acid decarboxylase . This results in the depletion of GTP and UTP nucleotide pools, affecting the synthesis of RNA and DNA .
Result of Action
The result of 6-AU’s action is the inhibition of growth in various microorganisms, including yeast . This is achieved through the depletion of intracellular GTP and UTP nucleotide pools, which diminishes transcription elongation . In yeast, 6-AU has been shown to induce transcription of the PUR5 gene, which encodes IMPDH-related enzymes .
Action Environment
It is known that 6-au enters saccharomyces cerevisiae cells through the uracil permease fur4 . This suggests that the presence and activity of specific transport proteins in the cell membrane may influence the efficacy of 6-AU.
Biochemical Analysis
Biochemical Properties
6-Azauracil plays a significant role in biochemical reactions by inhibiting the growth of various microorganisms. It achieves this by depleting intracellular guanosine triphosphate (GTP) and uridine triphosphate (UTP) nucleotide pools . The compound interacts with enzymes such as orotidine-5’-monophosphate decarboxylase (OMPdecase), inhibiting its activity and thereby affecting nucleotide biosynthesis . Additionally, this compound has been shown to inhibit the enzyme inosine monophosphate dehydrogenase (IMPDH), which is crucial for GTP biosynthesis .
Cellular Effects
This compound exerts various effects on cellular processes. In yeast cells, it has been observed to cause changes in nucleoside pools, leading to a reduction in GTP levels by inhibiting IMP dehydrogenase . This depletion of nucleotide pools affects transcription elongation and overall cell viability. Furthermore, this compound has been shown to induce transcriptional changes in genes involved in nucleotide metabolism, such as the PUR5 gene in Saccharomyces cerevisiae .
Molecular Mechanism
At the molecular level, this compound exerts its effects by inhibiting enzymes involved in nucleotide biosynthesis. It competes with substrates in the pyrimidine biosynthetic pathway, being transformed into 6-aza-UMP, a powerful competitive inhibitor of orotidylic acid decarboxylase . This inhibition leads to alterations in nucleotide pool levels, which in turn affects transcription elongation and other cellular processes. Additionally, this compound has been shown to induce changes in gene expression by affecting the activity of RNA polymerase II .
Temporal Effects in Laboratory Settings
In laboratory settings, the effects of this compound have been observed to change over time. The compound is known to be stable at room temperature and can be dissolved in ammonium hydroxide to yield a clear solution . Long-term exposure to this compound has been shown to cause diminished transcription of certain genes, such as the GAL1 gene in yeast . Additionally, mutants defective in histone methyltransferase genes have increased susceptibility to this compound treatment .
Dosage Effects in Animal Models
The effects of this compound vary with different dosages in animal models. In mice, high doses of this compound have been shown to induce profound hypnosis and weight loss, with repeated administration proving fatal at certain levels . In dogs, repeated administration of the compound caused anorexia, vomiting, diarrhea, emaciation, lethargy, and death . In monkeys, even massive doses failed to elicit toxic manifestations .
Metabolic Pathways
This compound is involved in the pyrimidine biosynthetic pathway, where it is transformed into 6-aza-UMP, a competitive inhibitor of orotidylic acid decarboxylase . This inhibition leads to alterations in nucleotide pool levels, affecting various metabolic processes. The compound also interacts with enzymes such as inosine monophosphate dehydrogenase, further influencing nucleotide metabolism .
Transport and Distribution
Within cells, this compound is transported and distributed through specific transporters and binding proteins. In yeast, it has been shown to enter cells through the uracil permease Fur4 . The compound’s distribution within tissues and cells affects its localization and accumulation, influencing its overall activity and function.
Subcellular Localization
This compound’s subcellular localization is influenced by its interactions with various biomolecules. In yeast, it has been observed to localize in the plasma membrane, where it interacts with the SNG1 protein to confer resistance to the compound . This localization is crucial for its inhibitory effects on nucleotide biosynthesis and transcription elongation.
Preparation Methods
Synthetic Routes and Reaction Conditions: The synthesis of 6-azauracil typically involves the cyclization of semicarbazone derivatives. One common method includes the cyclization of semicarbazone of glyoxylic acid using an excess of a trialkyl-silylation agent, followed by acid hydrolysis to yield this compound . Another method involves the reaction of silylated 6-azauracils with appropriate chloroethers to produce non-nucleoside derivatives .
Industrial Production Methods: Industrial production of this compound often employs similar synthetic routes but on a larger scale. The use of automated reactors and continuous flow systems can enhance the efficiency and yield of the synthesis process. The key steps include the preparation of semicarbazone intermediates, cyclization, and purification of the final product .
Chemical Reactions Analysis
Types of Reactions: 6-Azauracil undergoes various chemical reactions, including:
Substitution Reactions: Reaction with chloroethers to form non-nucleoside derivatives.
Cyclization Reactions: Formation of polyheterocyclic systems through reactions with electrophilic and nucleophilic reagents.
Common Reagents and Conditions:
Silylation Agents: Used in the initial steps of synthesis to protect functional groups.
Chloroethers: Employed in substitution reactions to introduce different substituents.
Electrophilic and Nucleophilic Reagents: Utilized in cyclization reactions to form complex heterocyclic structures.
Major Products:
Non-Nucleoside Derivatives: Formed through substitution reactions.
Polyheterocyclic Systems: Resulting from cyclization reactions with various reagents.
Scientific Research Applications
6-Azauracil has a wide range of applications in scientific research:
Chemistry: Used as a building block for the synthesis of complex heterocyclic compounds.
Biology: Investigated for its role in modulating transcription in yeast models.
Medicine: Explored for its antimicrobial, antiviral, and anticancer properties
Industry: Utilized in the development of new pharmaceuticals and agrochemicals.
Comparison with Similar Compounds
Uracil: A naturally occurring pyrimidine with similar structure but different biological activity.
6-Azauridine: A nucleoside analog with antiviral and anticancer properties.
5-Bromo-6-azauracil: A derivative with distinct fluorescence properties.
Uniqueness: 6-Azauracil is unique due to its ability to inhibit multiple enzymes in the nucleotide biosynthesis pathway, making it a potent growth inhibitor for various microorganisms.
Properties
IUPAC Name |
2H-1,2,4-triazine-3,5-dione | |
---|---|---|
Source | PubChem | |
URL | https://pubchem.ncbi.nlm.nih.gov | |
Description | Data deposited in or computed by PubChem | |
InChI |
InChI=1S/C3H3N3O2/c7-2-1-4-6-3(8)5-2/h1H,(H2,5,6,7,8) | |
Source | PubChem | |
URL | https://pubchem.ncbi.nlm.nih.gov | |
Description | Data deposited in or computed by PubChem | |
InChI Key |
SSPYSWLZOPCOLO-UHFFFAOYSA-N | |
Source | PubChem | |
URL | https://pubchem.ncbi.nlm.nih.gov | |
Description | Data deposited in or computed by PubChem | |
Canonical SMILES |
C1=NNC(=O)NC1=O | |
Source | PubChem | |
URL | https://pubchem.ncbi.nlm.nih.gov | |
Description | Data deposited in or computed by PubChem | |
Molecular Formula |
C3H3N3O2 | |
Source | PubChem | |
URL | https://pubchem.ncbi.nlm.nih.gov | |
Description | Data deposited in or computed by PubChem | |
DSSTOX Substance ID |
DTXSID2060042 | |
Record name | 1,2,4-Triazine-3,5(2H,4H)-dione | |
Source | EPA DSSTox | |
URL | https://comptox.epa.gov/dashboard/DTXSID2060042 | |
Description | DSSTox provides a high quality public chemistry resource for supporting improved predictive toxicology. | |
Molecular Weight |
113.08 g/mol | |
Source | PubChem | |
URL | https://pubchem.ncbi.nlm.nih.gov | |
Description | Data deposited in or computed by PubChem | |
CAS No. |
461-89-2 | |
Record name | 1,2,4-Triazine-3,5(2H,4H)-dione | |
Source | CAS Common Chemistry | |
URL | https://commonchemistry.cas.org/detail?cas_rn=461-89-2 | |
Description | CAS Common Chemistry is an open community resource for accessing chemical information. Nearly 500,000 chemical substances from CAS REGISTRY cover areas of community interest, including common and frequently regulated chemicals, and those relevant to high school and undergraduate chemistry classes. This chemical information, curated by our expert scientists, is provided in alignment with our mission as a division of the American Chemical Society. | |
Explanation | The data from CAS Common Chemistry is provided under a CC-BY-NC 4.0 license, unless otherwise stated. | |
Record name | Azauracil | |
Source | ChemIDplus | |
URL | https://pubchem.ncbi.nlm.nih.gov/substance/?source=chemidplus&sourceid=0000461892 | |
Description | ChemIDplus is a free, web search system that provides access to the structure and nomenclature authority files used for the identification of chemical substances cited in National Library of Medicine (NLM) databases, including the TOXNET system. | |
Record name | 6-Azauracil | |
Source | DTP/NCI | |
URL | https://dtp.cancer.gov/dtpstandard/servlet/dwindex?searchtype=NSC&outputformat=html&searchlist=3425 | |
Description | The NCI Development Therapeutics Program (DTP) provides services and resources to the academic and private-sector research communities worldwide to facilitate the discovery and development of new cancer therapeutic agents. | |
Explanation | Unless otherwise indicated, all text within NCI products is free of copyright and may be reused without our permission. Credit the National Cancer Institute as the source. | |
Record name | 1,2,4-Triazine-3,5(2H,4H)-dione | |
Source | EPA Chemicals under the TSCA | |
URL | https://www.epa.gov/chemicals-under-tsca | |
Description | EPA Chemicals under the Toxic Substances Control Act (TSCA) collection contains information on chemicals and their regulations under TSCA, including non-confidential content from the TSCA Chemical Substance Inventory and Chemical Data Reporting. | |
Record name | 1,2,4-Triazine-3,5(2H,4H)-dione | |
Source | EPA DSSTox | |
URL | https://comptox.epa.gov/dashboard/DTXSID2060042 | |
Description | DSSTox provides a high quality public chemistry resource for supporting improved predictive toxicology. | |
Record name | 1,2,4-triazine-3,5-diol | |
Source | European Chemicals Agency (ECHA) | |
URL | https://echa.europa.eu/substance-information/-/substanceinfo/100.006.654 | |
Description | The European Chemicals Agency (ECHA) is an agency of the European Union which is the driving force among regulatory authorities in implementing the EU's groundbreaking chemicals legislation for the benefit of human health and the environment as well as for innovation and competitiveness. | |
Explanation | Use of the information, documents and data from the ECHA website is subject to the terms and conditions of this Legal Notice, and subject to other binding limitations provided for under applicable law, the information, documents and data made available on the ECHA website may be reproduced, distributed and/or used, totally or in part, for non-commercial purposes provided that ECHA is acknowledged as the source: "Source: European Chemicals Agency, http://echa.europa.eu/". Such acknowledgement must be included in each copy of the material. ECHA permits and encourages organisations and individuals to create links to the ECHA website under the following cumulative conditions: Links can only be made to webpages that provide a link to the Legal Notice page. | |
Record name | 6-AZAURACIL | |
Source | FDA Global Substance Registration System (GSRS) | |
URL | https://gsrs.ncats.nih.gov/ginas/app/beta/substances/I14TWN70LR | |
Description | The FDA Global Substance Registration System (GSRS) enables the efficient and accurate exchange of information on what substances are in regulated products. Instead of relying on names, which vary across regulatory domains, countries, and regions, the GSRS knowledge base makes it possible for substances to be defined by standardized, scientific descriptions. | |
Explanation | Unless otherwise noted, the contents of the FDA website (www.fda.gov), both text and graphics, are not copyrighted. They are in the public domain and may be republished, reprinted and otherwise used freely by anyone without the need to obtain permission from FDA. Credit to the U.S. Food and Drug Administration as the source is appreciated but not required. | |
Retrosynthesis Analysis
AI-Powered Synthesis Planning: Our tool employs the Template_relevance Pistachio, Template_relevance Bkms_metabolic, Template_relevance Pistachio_ringbreaker, Template_relevance Reaxys, Template_relevance Reaxys_biocatalysis model, leveraging a vast database of chemical reactions to predict feasible synthetic routes.
One-Step Synthesis Focus: Specifically designed for one-step synthesis, it provides concise and direct routes for your target compounds, streamlining the synthesis process.
Accurate Predictions: Utilizing the extensive PISTACHIO, BKMS_METABOLIC, PISTACHIO_RINGBREAKER, REAXYS, REAXYS_BIOCATALYSIS database, our tool offers high-accuracy predictions, reflecting the latest in chemical research and data.
Strategy Settings
Precursor scoring | Relevance Heuristic |
---|---|
Min. plausibility | 0.01 |
Model | Template_relevance |
Template Set | Pistachio/Bkms_metabolic/Pistachio_ringbreaker/Reaxys/Reaxys_biocatalysis |
Top-N result to add to graph | 6 |
Feasible Synthetic Routes
Q1: What is the primary mechanism of action of 6-azauracil?
A1: this compound exerts its biological effects primarily by disrupting pyrimidine nucleotide biosynthesis. It achieves this by inhibiting uracil phosphoribosyltransferase, a key enzyme in the pyrimidine salvage pathway. [] This enzyme typically converts uracil to uridine monophosphate (UMP), a precursor for RNA synthesis. By inhibiting this conversion, this compound effectively starves cells of the pyrimidine nucleotides crucial for RNA production. [, ]
Q2: How does the inhibition of uracil phosphoribosyltransferase by this compound impact cells?
A2: Inhibition of uracil phosphoribosyltransferase leads to a depletion of UMP, ultimately hindering RNA synthesis. [, ] This disruption in RNA synthesis has a cascading effect on various cellular processes, leading to growth inhibition and cell death. [, , ]
Q3: Are there other enzymes involved in pyrimidine metabolism that are affected by this compound?
A3: Yes, in addition to inhibiting uracil phosphoribosyltransferase, this compound can be metabolized to 6-azauridine monophosphate, a potent inhibitor of orotate monophosphate decarboxylase. [, ] This enzyme is involved in the de novo synthesis of pyrimidine nucleotides, further contributing to the overall disruption of pyrimidine metabolism.
Q4: Does this compound directly influence beta-alanine metabolism?
A4: Research indicates that this compound indirectly affects beta-alanine metabolism in rats by inhibiting enzymes responsible for its catabolism, specifically beta-alanine-oxoglutarate aminotransferase and D-3-aminoisobutyrate-pyruvate aminotransferase. [] This inhibition leads to an accumulation of beta-alanine in the liver, though the exact mechanism behind this enzyme inhibition remains to be fully elucidated. []
Q5: What is the molecular formula and molecular weight of this compound?
A5: The molecular formula of this compound is C3H3N3O2, and its molecular weight is 113.08 g/mol.
Q6: What spectroscopic techniques have been employed to characterize this compound and its derivatives?
A6: Several spectroscopic methods have been used to elucidate the structural features of this compound and its derivatives. These include:* Infrared Spectroscopy (IR): This technique provides information about the functional groups present in the molecule, such as carbonyl and amine groups. [, ]* Nuclear Magnetic Resonance (NMR) Spectroscopy (1H and 13C): NMR spectroscopy helps determine the structure and purity of the synthesized compounds, providing details about the arrangement of hydrogen and carbon atoms. [, ]* Mass Spectrometry (MS): MS is used to determine the molecular weight and fragmentation patterns of the molecule, aiding in structure confirmation and identification. []
Q7: Have computational methods been used to study this compound?
A7: Yes, computational chemistry has played a role in understanding this compound. For instance, studies have employed quantum chemical calculations to investigate the water-assisted intramolecular proton-transfer processes of 5- and this compound in aqueous solutions. [] This involved using methods like MP2 and CCSD(T) to determine the optimized geometries and energies of various tautomeric forms of the molecule in the presence of explicit water molecules and a continuum solvent model. []
Q8: How does the structure of this compound relate to its biological activity?
A8: Modifications to the this compound structure significantly impact its activity. For example, benzylation at the N-1 position, mimicking the ribose phosphate attachment site in natural nucleotides, enhances anticoccidial activity. [] Introducing electron-withdrawing substituents on the benzyl group further augments this effect. [] Studies on derivatives with phenyl sulfide and phenyl sulfone side chains at the N-1 position reveal a substantial potency increase against coccidial infections, highlighting the importance of specific structural features for activity. []
Q9: What types of in vivo models have been used to evaluate the efficacy of this compound?
A10: Various in vivo models have been employed to assess the efficacy of this compound and its derivatives. These include:* Avian Coccidiosis Model: Studies have demonstrated the effectiveness of this compound derivatives in preventing coccidial infections in chickens. [, , , ] Notably, modifications to the N-1 substituent drastically influence potency, with some derivatives exhibiting up to a 4000-fold increase in potency compared to this compound. [] * Plant Models: Researchers have utilized tobacco tissue cultures to examine the growth inhibitory effects of this compound and its derivatives, drawing parallels to their effects on nucleic acid synthesis in mammalian systems. [] Additionally, studies on cotton ovules grown in vitro have revealed that this compound induces boron deficiency-like symptoms, providing insights into the potential role of this compound in plant metabolism. []
Q10: Has this compound been investigated in clinical trials?
A11: While this compound has been explored in clinical settings, its use has been limited due to concerns over its toxicity, particularly its effects on the central nervous system. [] Early clinical studies focused on its potential as an anticancer agent, but these were hampered by its adverse effects. []
Q11: Have any resistance mechanisms to this compound been identified?
A12: Yes, studies using plant cells have identified that resistance to this compound can arise from the loss of uracil phosphoribosyltransferase activity. [] This suggests that cells lacking this enzyme can bypass the metabolic block imposed by this compound, enabling them to maintain pyrimidine nucleotide levels and survive.
Q12: What are the primary safety concerns associated with this compound?
A13: The primary safety concerns surrounding this compound relate to its neurological effects. Clinical studies have reported that this compound can induce a range of central nervous system disturbances, including lethargy, sensory and motor abnormalities, and electroencephalographic changes. [] Children appear to be more susceptible to these neurological effects than adults. []
Disclaimer and Information on In-Vitro Research Products
Please be aware that all articles and product information presented on BenchChem are intended solely for informational purposes. The products available for purchase on BenchChem are specifically designed for in-vitro studies, which are conducted outside of living organisms. In-vitro studies, derived from the Latin term "in glass," involve experiments performed in controlled laboratory settings using cells or tissues. It is important to note that these products are not categorized as medicines or drugs, and they have not received approval from the FDA for the prevention, treatment, or cure of any medical condition, ailment, or disease. We must emphasize that any form of bodily introduction of these products into humans or animals is strictly prohibited by law. It is essential to adhere to these guidelines to ensure compliance with legal and ethical standards in research and experimentation.