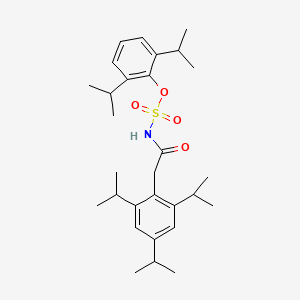
Avasimibe
Overview
Description
Avasimibe is a compound known for its ability to inhibit sterol O-acyltransferases (SOAT1 and SOAT2), enzymes involved in the metabolism and catabolism of cholesterol . It was discovered by Parke-Davis (later Pfizer) and initially developed as a potential lipid-lowering agent and treatment for atherosclerosis . Although its development was halted due to interactions with other medicines and lack of favorable effects on atherosclerosis, this compound has gained renewed interest for its potential antitumor properties .
Mechanism of Action
Target of Action
Avasimibe primarily targets the enzymes sterol O-acyltransferases (SOAT1 and SOAT2, also known as ACAT1 and ACAT2) . These enzymes play a crucial role in the metabolism and catabolism of cholesterol . ACAT1 has been found to be widely involved in tumor initiation and progression .
Mode of Action
This compound is a potent inhibitor of ACAT1 . It inhibits the conversion of excessive cholesterol to less toxic cholesteryl esters . This compound is also a potent activator of the pregnane X receptor, an indirect inducer of CYP3A4 and P-glycoprotein, and a potent inhibitor of several cytochrome P450 isoenzymes, including CYP1A2, CYP2C9, and CYP2C19 .
Biochemical Pathways
This compound affects multiple biochemical pathways. It suppresses the expression of CDK2, cyclin E1, CDK4, cyclin D, CDK1, cyclin B1, Aurora A, and PLK1, while inducing the expression of p53, p21, p27, and GADD45A . This results in the induction of mitochondria-dependent apoptosis in glioblastoma cells, associated with arresting the cell cycle at G0/G1 phase and G2/M phase by regulating the p53/p21 pathway, p53/GADD45A, and Aurora A/PLK1 signaling pathways .
Pharmacokinetics
This compound is metabolized in the liver, primarily by the CYP3A4 and 2C9 enzymes . The elimination half-life of this compound is between 15 and 24 hours . The compound is predominantly excreted in feces, with less than 2% excreted in urine . This compound is better absorbed when taken with food, especially with a high-fat meal, as reflected by increases in its peak serum concentration and area under the curve (AUC) .
Result of Action
This compound has been shown to have anticancer effects. It dose-dependently inhibits the proliferation of certain cancer cells, decreases DNA synthesis, and inhibits the colony formation of tumor cells . It also dose-dependently increases the apoptotic rate of tumor cells, decreases the mitochondrial membrane potential, induces the activity of caspase-3/7, and increases the protein expression of cleaved caspase-9, cleaved PARP, and Bax .
Action Environment
The action, efficacy, and stability of this compound can be influenced by various environmental factors. For instance, the absorption of this compound is enhanced when taken with food, particularly a high-fat meal . .
Biochemical Analysis
Biochemical Properties
Avasimibe is a potent activator of the pregnane X receptor and, consequently, an indirect inducer of CYP3A4 and P-glycoprotein . It is also a potent inhibitor of several cytochrome P450 isoenzymes, including CYP1A2, CYP2C9, and CYP2C19 . These interactions with enzymes and proteins play a crucial role in its biochemical reactions.
Cellular Effects
This compound has been found to have significant effects on various types of cells. For instance, it has been shown to suppress tumor growth and trigger G1 phase arrest in glioblastoma cells . It also dose-dependently increased the apoptotic rate of tumor cells, decreased the mitochondrial membrane potential, induced the activity of caspase-3/7, and increased the protein expression of cleaved caspase-9, cleaved PARP, and Bax in U251 and U87 cells .
Molecular Mechanism
This compound exerts its effects at the molecular level through various mechanisms. It induces mitochondria-dependent apoptosis in glioblastoma cells, which is associated with arresting the cell cycle at G0/G1 phase and G2/M phase by regulating the p53/p21 pathway, p53/GADD45A, and Aurora A/PLK1 signaling pathways .
Temporal Effects in Laboratory Settings
In laboratory settings, this compound has been shown to have significant temporal effects. For instance, it has been found to suppress tumor growth and trigger G1 phase arrest over time . Moreover, the expression of the cell cycle-related proteins CDK2/4/6, Cyclin D1, and Cyclin A1+A2 was significantly increased, and p21 expression was decreased after this compound treatment .
Dosage Effects in Animal Models
In animal models, the effects of this compound vary with different dosages. For example, in beagle dogs, significant toxicologic findings were restricted to the higher doses (≥300 mg/kg) and included emesis, fecal consistency changes, salivation, body weight loss, microscopic and clinical pathologic evidence of hepatic toxicity, and red blood cell (RBC) morphology changes .
Preparation Methods
Avasimibe was the result of a rational drug design process aimed at obtaining orally bioavailable, water-soluble ACAT inhibitors . The synthetic route involves the reaction of 2,6-diisopropylphenol with 2,4,6-triisopropylbenzoyl chloride to form the intermediate, which is then reacted with sulfamoyl chloride to yield this compound . The reaction conditions typically involve the use of organic solvents and controlled temperatures to ensure the stability and purity of the final product .
Chemical Reactions Analysis
Avasimibe undergoes various chemical reactions, including:
Oxidation: this compound can be oxidized under specific conditions to form corresponding oxidized products.
Reduction: It can also undergo reduction reactions, although these are less common.
Substitution: This compound can participate in substitution reactions, particularly involving its functional groups. Common reagents used in these reactions include oxidizing agents like hydrogen peroxide and reducing agents like sodium borohydride. The major products formed depend on the specific reaction conditions and reagents used.
Scientific Research Applications
Comparison with Similar Compounds
Avasimibe is unique in its dual role as a sterol O-acyltransferase inhibitor and a pregnane X receptor activator . Similar compounds include:
F12511: A sterol O-acyltransferase inhibitor with potential antitumor properties, similar to this compound.
Rifampicin: Although primarily an antibiotic, rifampicin shares a similar spectrum of CYP induction and inhibition with this compound.
This compound’s unique combination of enzyme inhibition and receptor activation sets it apart from these compounds, making it a valuable tool for both research and potential therapeutic applications .
Properties
IUPAC Name |
[2,6-di(propan-2-yl)phenyl] N-[2-[2,4,6-tri(propan-2-yl)phenyl]acetyl]sulfamate | |
---|---|---|
Source | PubChem | |
URL | https://pubchem.ncbi.nlm.nih.gov | |
Description | Data deposited in or computed by PubChem | |
InChI |
InChI=1S/C29H43NO4S/c1-17(2)22-14-25(20(7)8)27(26(15-22)21(9)10)16-28(31)30-35(32,33)34-29-23(18(3)4)12-11-13-24(29)19(5)6/h11-15,17-21H,16H2,1-10H3,(H,30,31) | |
Source | PubChem | |
URL | https://pubchem.ncbi.nlm.nih.gov | |
Description | Data deposited in or computed by PubChem | |
InChI Key |
PTQXTEKSNBVPQJ-UHFFFAOYSA-N | |
Source | PubChem | |
URL | https://pubchem.ncbi.nlm.nih.gov | |
Description | Data deposited in or computed by PubChem | |
Canonical SMILES |
CC(C)C1=C(C(=CC=C1)C(C)C)OS(=O)(=O)NC(=O)CC2=C(C=C(C=C2C(C)C)C(C)C)C(C)C | |
Source | PubChem | |
URL | https://pubchem.ncbi.nlm.nih.gov | |
Description | Data deposited in or computed by PubChem | |
Molecular Formula |
C29H43NO4S | |
Source | PubChem | |
URL | https://pubchem.ncbi.nlm.nih.gov | |
Description | Data deposited in or computed by PubChem | |
DSSTOX Substance ID |
DTXSID80168117 | |
Record name | Avasimibe | |
Source | EPA DSSTox | |
URL | https://comptox.epa.gov/dashboard/DTXSID80168117 | |
Description | DSSTox provides a high quality public chemistry resource for supporting improved predictive toxicology. | |
Molecular Weight |
501.7 g/mol | |
Source | PubChem | |
URL | https://pubchem.ncbi.nlm.nih.gov | |
Description | Data deposited in or computed by PubChem | |
CAS No. |
166518-60-1 | |
Record name | Avasimibe | |
Source | CAS Common Chemistry | |
URL | https://commonchemistry.cas.org/detail?cas_rn=166518-60-1 | |
Description | CAS Common Chemistry is an open community resource for accessing chemical information. Nearly 500,000 chemical substances from CAS REGISTRY cover areas of community interest, including common and frequently regulated chemicals, and those relevant to high school and undergraduate chemistry classes. This chemical information, curated by our expert scientists, is provided in alignment with our mission as a division of the American Chemical Society. | |
Explanation | The data from CAS Common Chemistry is provided under a CC-BY-NC 4.0 license, unless otherwise stated. | |
Record name | Avasimibe [USAN:INN] | |
Source | ChemIDplus | |
URL | https://pubchem.ncbi.nlm.nih.gov/substance/?source=chemidplus&sourceid=0166518601 | |
Description | ChemIDplus is a free, web search system that provides access to the structure and nomenclature authority files used for the identification of chemical substances cited in National Library of Medicine (NLM) databases, including the TOXNET system. | |
Record name | Avasimibe | |
Source | DrugBank | |
URL | https://www.drugbank.ca/drugs/DB06442 | |
Description | The DrugBank database is a unique bioinformatics and cheminformatics resource that combines detailed drug (i.e. chemical, pharmacological and pharmaceutical) data with comprehensive drug target (i.e. sequence, structure, and pathway) information. | |
Explanation | Creative Common's Attribution-NonCommercial 4.0 International License (http://creativecommons.org/licenses/by-nc/4.0/legalcode) | |
Record name | Avasimibe | |
Source | EPA DSSTox | |
URL | https://comptox.epa.gov/dashboard/DTXSID80168117 | |
Description | DSSTox provides a high quality public chemistry resource for supporting improved predictive toxicology. | |
Record name | AVASIMIBE | |
Source | FDA Global Substance Registration System (GSRS) | |
URL | https://gsrs.ncats.nih.gov/ginas/app/beta/substances/28LQ20T5RC | |
Description | The FDA Global Substance Registration System (GSRS) enables the efficient and accurate exchange of information on what substances are in regulated products. Instead of relying on names, which vary across regulatory domains, countries, and regions, the GSRS knowledge base makes it possible for substances to be defined by standardized, scientific descriptions. | |
Explanation | Unless otherwise noted, the contents of the FDA website (www.fda.gov), both text and graphics, are not copyrighted. They are in the public domain and may be republished, reprinted and otherwise used freely by anyone without the need to obtain permission from FDA. Credit to the U.S. Food and Drug Administration as the source is appreciated but not required. | |
Retrosynthesis Analysis
AI-Powered Synthesis Planning: Our tool employs the Template_relevance Pistachio, Template_relevance Bkms_metabolic, Template_relevance Pistachio_ringbreaker, Template_relevance Reaxys, Template_relevance Reaxys_biocatalysis model, leveraging a vast database of chemical reactions to predict feasible synthetic routes.
One-Step Synthesis Focus: Specifically designed for one-step synthesis, it provides concise and direct routes for your target compounds, streamlining the synthesis process.
Accurate Predictions: Utilizing the extensive PISTACHIO, BKMS_METABOLIC, PISTACHIO_RINGBREAKER, REAXYS, REAXYS_BIOCATALYSIS database, our tool offers high-accuracy predictions, reflecting the latest in chemical research and data.
Strategy Settings
Precursor scoring | Relevance Heuristic |
---|---|
Min. plausibility | 0.01 |
Model | Template_relevance |
Template Set | Pistachio/Bkms_metabolic/Pistachio_ringbreaker/Reaxys/Reaxys_biocatalysis |
Top-N result to add to graph | 6 |
Feasible Synthetic Routes
Disclaimer and Information on In-Vitro Research Products
Please be aware that all articles and product information presented on BenchChem are intended solely for informational purposes. The products available for purchase on BenchChem are specifically designed for in-vitro studies, which are conducted outside of living organisms. In-vitro studies, derived from the Latin term "in glass," involve experiments performed in controlled laboratory settings using cells or tissues. It is important to note that these products are not categorized as medicines or drugs, and they have not received approval from the FDA for the prevention, treatment, or cure of any medical condition, ailment, or disease. We must emphasize that any form of bodily introduction of these products into humans or animals is strictly prohibited by law. It is essential to adhere to these guidelines to ensure compliance with legal and ethical standards in research and experimentation.