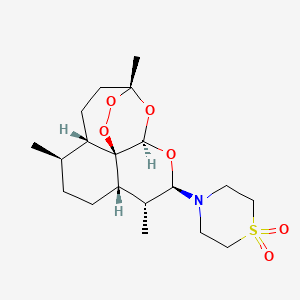
Artemisone
Overview
Description
Artemisone is a second-generation semi-synthetic derivative of artemisinin, a compound extracted from the plant Artemisia annua, commonly known as sweet wormwood. This compound was developed to enhance the efficacy and reduce the toxicity of artemisinin derivatives, primarily for the treatment of malaria. It has shown promising results in preclinical and clinical studies due to its potent antimalarial activity and improved pharmacokinetic properties .
Mechanism of Action
Target of Action
Artemisone, like other artemisinin derivatives, primarily targets the malaria parasite Plasmodium falciparum . It has also been found to be active against cancer cells and has shown to reduce CD4+ and CD8+ T cell inflammatory responses .
Mode of Action
This metabolite undergoes cleavage of its endoperoxide ring inside the erythrocytes . The endoperoxide 1,2,4-trioxane ring in artemisinin and its derivatives is responsible for their antimalarial properties .
Biochemical Pathways
Any proposed pathway for the toxicity of artemisinins on plasmodia must incorporate the direct or indirect action of the endoperoxide moiety on a putative molecular target . The major mechanisms of action of artemisinins may be due to toxic-free radicals generated by an endoperoxide moiety, cell cycle arrest, induction of apoptosis, and inhibition of tumor angiogenesis .
Pharmacokinetics
This compound has improved pharmacokinetics compared to other artemisinin derivatives . The controlled release of this compound from biodegradable polymers has been studied in a mouse model, showing significant amounts of this compound released throughout at least ten days .
Result of Action
This compound has been found to stall tumor cell proliferation through cell cycle arrest mediated by reactive oxygen species (ROS) . In a study, this compound alone was found to effect cell cycle arrest 30-fold more than artemisinin . This compound effected G1-selective blockade, whereas artemisinin resulted in a general arrest in all phases .
Action Environment
The action of this compound can be influenced by various environmental factors. For instance, the efficacy of this compound can be enhanced when combined with other drugs. Combinations of this compound with synthetic chemodrugs have been studied to enhance the latter’s efficacy . The emergence of artemisinins’ nano-based formulations in combination with chemodrugs to enhance drug bioavailability and targeting as well as immunotherapy is also being reviewed .
Biochemical Analysis
Biochemical Properties
Artemisone plays a significant role in biochemical reactions, particularly in its interaction with heme and iron. The compound’s endoperoxide bridge reacts with ferrous iron, leading to the generation of reactive oxygen species (ROS). These ROS cause oxidative damage to biomolecules, including proteins and lipids, which is crucial for its antimalarial activity . This compound also interacts with the sarcoplasmic/endoplasmic reticulum calcium ATPase (SERCA), disrupting calcium homeostasis in the parasite .
Cellular Effects
This compound exerts various effects on different types of cells and cellular processes. In cancer cells, this compound induces cell cycle arrest, particularly at the G1 phase, and promotes apoptosis through the generation of ROS . It also affects cell signaling pathways, including the PI3K-Akt and TGF-β pathways, which are involved in cell proliferation and apoptosis . Additionally, this compound influences gene expression and cellular metabolism, leading to the inhibition of tumor growth and metastasis .
Molecular Mechanism
The molecular mechanism of this compound involves its interaction with heme and iron, leading to the formation of ROS that damage essential biomolecules in the parasite . This compound also inhibits the activity of SERCA, disrupting calcium homeostasis and leading to cell death . Furthermore, this compound’s ability to generate ROS plays a crucial role in its anticancer activity by inducing oxidative stress and apoptosis in cancer cells .
Temporal Effects in Laboratory Settings
In laboratory settings, the effects of this compound have been observed to change over time. The compound exhibits stability and retains its efficacy over extended periods . Long-term studies have shown that this compound maintains its antimalarial activity without significant degradation . Additionally, in vitro and in vivo studies have demonstrated that this compound’s effects on cellular function, including its antiproliferative and pro-apoptotic activities, persist over time .
Dosage Effects in Animal Models
The effects of this compound vary with different dosages in animal models. At lower doses, this compound effectively inhibits parasite growth and reduces parasitemia . At higher doses, this compound can exhibit toxic effects, including hepatotoxicity and neurotoxicity . Studies have identified threshold doses that balance efficacy and safety, ensuring optimal therapeutic outcomes without adverse effects .
Metabolic Pathways
This compound is involved in several metabolic pathways, including those related to its activation and detoxification. The compound undergoes biotransformation in the liver, where it is metabolized by cytochrome P450 enzymes . This metabolism leads to the formation of active metabolites that contribute to its therapeutic effects . This compound also affects metabolic flux and metabolite levels, influencing cellular energy production and redox balance .
Transport and Distribution
This compound is transported and distributed within cells and tissues through passive diffusion and active transport mechanisms . The compound interacts with transporters and binding proteins, facilitating its uptake and accumulation in target cells . This compound’s distribution is influenced by its lipophilicity, allowing it to penetrate cellular membranes and reach intracellular compartments .
Subcellular Localization
This compound localizes to specific subcellular compartments, including the endoplasmic reticulum and mitochondria . This localization is mediated by targeting signals and post-translational modifications that direct this compound to these organelles . The subcellular localization of this compound is crucial for its activity, as it allows the compound to interact with key biomolecules and exert its therapeutic effects .
Preparation Methods
Synthetic Routes and Reaction Conditions: The synthesis of artemisone involves several steps, starting from dihydroartemisinin, a derivative of artemisinin. The key steps include:
Oxidation: Dihydroartemisinin is oxidized to form an intermediate compound.
Thiomorpholine Introduction: The intermediate is then reacted with thiomorpholine to introduce the thiomorpholine moiety.
Purification: The final product, this compound, is purified using chromatographic techniques.
Industrial Production Methods: Industrial production of this compound follows similar synthetic routes but on a larger scale. The process involves:
Bulk Synthesis: Large-scale oxidation and thiomorpholine introduction reactions.
Crystallization: The product is crystallized to ensure purity.
Quality Control: Rigorous quality control measures are implemented to ensure the consistency and efficacy of the final product.
Chemical Reactions Analysis
Types of Reactions: Artemisone undergoes various chemical reactions, including:
Oxidation: this compound can be oxidized to form different derivatives.
Reduction: Reduction reactions can modify the peroxide bridge in this compound.
Substitution: Substitution reactions can introduce different functional groups into the this compound molecule.
Common Reagents and Conditions:
Oxidizing Agents: Hydrogen peroxide, m-chloroperbenzoic acid.
Reducing Agents: Sodium borohydride, lithium aluminum hydride.
Solvents: Dichloromethane, ethanol, methanol.
Major Products: The major products formed from these reactions include various artemisinin derivatives with modified pharmacological properties .
Scientific Research Applications
Artemisone has a wide range of scientific research applications:
Comparison with Similar Compounds
Artemisinin: The parent compound from which artemisone is derived.
Artesunate: Another semi-synthetic derivative of artemisinin with similar antimalarial properties.
Dihydroartemisinin: A direct derivative of artemisinin used in combination therapies.
Comparison: this compound is unique among artemisinin derivatives due to its improved pharmacokinetic properties and reduced toxicity. Unlike artemisinin, which has poor bioavailability, this compound is more readily absorbed by the body. Compared to artesunate and dihydroartemisinin, this compound has shown greater efficacy in preclinical studies and a lower incidence of adverse effects .
This compound represents a significant advancement in the treatment of malaria and other parasitic infections, offering improved efficacy and safety profiles compared to its predecessors.
Properties
IUPAC Name |
4-[(1R,4S,5R,8S,9R,10R,12R,13R)-1,5,9-trimethyl-11,14,15,16-tetraoxatetracyclo[10.3.1.04,13.08,13]hexadecan-10-yl]-1,4-thiazinane 1,1-dioxide | |
---|---|---|
Source | PubChem | |
URL | https://pubchem.ncbi.nlm.nih.gov | |
Description | Data deposited in or computed by PubChem | |
InChI |
InChI=1S/C19H31NO6S/c1-12-4-5-15-13(2)16(20-8-10-27(21,22)11-9-20)23-17-19(15)14(12)6-7-18(3,24-17)25-26-19/h12-17H,4-11H2,1-3H3/t12-,13-,14+,15+,16-,17-,18-,19-/m1/s1 | |
Source | PubChem | |
URL | https://pubchem.ncbi.nlm.nih.gov | |
Description | Data deposited in or computed by PubChem | |
InChI Key |
FDMUNKXWYMSZIR-NQWKWHCYSA-N | |
Source | PubChem | |
URL | https://pubchem.ncbi.nlm.nih.gov | |
Description | Data deposited in or computed by PubChem | |
Canonical SMILES |
CC1CCC2C(C(OC3C24C1CCC(O3)(OO4)C)N5CCS(=O)(=O)CC5)C | |
Source | PubChem | |
URL | https://pubchem.ncbi.nlm.nih.gov | |
Description | Data deposited in or computed by PubChem | |
Isomeric SMILES |
C[C@@H]1CC[C@H]2[C@H]([C@@H](O[C@H]3[C@@]24[C@H]1CC[C@](O3)(OO4)C)N5CCS(=O)(=O)CC5)C | |
Source | PubChem | |
URL | https://pubchem.ncbi.nlm.nih.gov | |
Description | Data deposited in or computed by PubChem | |
Molecular Formula |
C19H31NO6S | |
Source | PubChem | |
URL | https://pubchem.ncbi.nlm.nih.gov | |
Description | Data deposited in or computed by PubChem | |
Molecular Weight |
401.5 g/mol | |
Source | PubChem | |
URL | https://pubchem.ncbi.nlm.nih.gov | |
Description | Data deposited in or computed by PubChem | |
CAS No. |
255730-18-8 | |
Record name | Artemisone | |
Source | CAS Common Chemistry | |
URL | https://commonchemistry.cas.org/detail?cas_rn=255730-18-8 | |
Description | CAS Common Chemistry is an open community resource for accessing chemical information. Nearly 500,000 chemical substances from CAS REGISTRY cover areas of community interest, including common and frequently regulated chemicals, and those relevant to high school and undergraduate chemistry classes. This chemical information, curated by our expert scientists, is provided in alignment with our mission as a division of the American Chemical Society. | |
Explanation | The data from CAS Common Chemistry is provided under a CC-BY-NC 4.0 license, unless otherwise stated. | |
Record name | Artemisone | |
Source | DrugBank | |
URL | https://www.drugbank.ca/drugs/DB16097 | |
Description | The DrugBank database is a unique bioinformatics and cheminformatics resource that combines detailed drug (i.e. chemical, pharmacological and pharmaceutical) data with comprehensive drug target (i.e. sequence, structure, and pathway) information. | |
Explanation | Creative Common's Attribution-NonCommercial 4.0 International License (http://creativecommons.org/licenses/by-nc/4.0/legalcode) | |
Record name | ARTEMISONE | |
Source | FDA Global Substance Registration System (GSRS) | |
URL | https://gsrs.ncats.nih.gov/ginas/app/beta/substances/796LMW5BUZ | |
Description | The FDA Global Substance Registration System (GSRS) enables the efficient and accurate exchange of information on what substances are in regulated products. Instead of relying on names, which vary across regulatory domains, countries, and regions, the GSRS knowledge base makes it possible for substances to be defined by standardized, scientific descriptions. | |
Explanation | Unless otherwise noted, the contents of the FDA website (www.fda.gov), both text and graphics, are not copyrighted. They are in the public domain and may be republished, reprinted and otherwise used freely by anyone without the need to obtain permission from FDA. Credit to the U.S. Food and Drug Administration as the source is appreciated but not required. | |
Retrosynthesis Analysis
AI-Powered Synthesis Planning: Our tool employs the Template_relevance Pistachio, Template_relevance Bkms_metabolic, Template_relevance Pistachio_ringbreaker, Template_relevance Reaxys, Template_relevance Reaxys_biocatalysis model, leveraging a vast database of chemical reactions to predict feasible synthetic routes.
One-Step Synthesis Focus: Specifically designed for one-step synthesis, it provides concise and direct routes for your target compounds, streamlining the synthesis process.
Accurate Predictions: Utilizing the extensive PISTACHIO, BKMS_METABOLIC, PISTACHIO_RINGBREAKER, REAXYS, REAXYS_BIOCATALYSIS database, our tool offers high-accuracy predictions, reflecting the latest in chemical research and data.
Strategy Settings
Precursor scoring | Relevance Heuristic |
---|---|
Min. plausibility | 0.01 |
Model | Template_relevance |
Template Set | Pistachio/Bkms_metabolic/Pistachio_ringbreaker/Reaxys/Reaxys_biocatalysis |
Top-N result to add to graph | 6 |
Feasible Synthetic Routes
ANone: While the exact mechanism of action remains debated, Artemisone is believed to function similarly to other artemisinin derivatives. A key aspect involves its interaction with iron, leading to the generation of reactive oxygen species (ROS) within the parasite. These ROS are thought to cause damage to vital parasite proteins and disrupt essential biological processes [, ]. One proposed target is the parasite's SERCA PfATP6, a calcium ATPase involved in calcium homeostasis []. This compound has been shown to inhibit PfATP6, potentially disrupting calcium signaling and contributing to parasite death [, ].
ANone: Yes, research has demonstrated that this compound, upon activation by iron(II)-heme, effectively generates covalent heme-drug adducts []. This alkylating ability is consistent with other artemisinin derivatives and is thought to be a significant factor in its antimalarial activity.
ANone: this compound has the molecular formula C19H31NO6S and a molecular weight of 401.53 g/mol [].
ANone: While specific spectroscopic data is not provided in the provided research articles, it's important to note that this compound's structure has been confirmed through various analytical techniques, including X-ray crystallography for its derivatives [].
ANone: While the provided research papers don't delve into detailed computational modeling of this compound, they highlight the potential for this approach. For example, understanding the interaction of this compound with PfATP6 through molecular docking simulations could provide further insights into its mechanism of action. Additionally, quantitative structure-activity relationship (QSAR) studies could be valuable for optimizing this compound's properties, as explored with its derivatives [].
ANone: this compound, as a 10-alkylamino artemisinin derivative, possesses structural features crucial for its activity. The presence of the endoperoxide bridge is essential for its interaction with iron and subsequent generation of ROS [, ]. Modifications at the C-10 position, specifically the amino group, play a crucial role in its potency and distinguish it from other artemisinin derivatives [, ]. Studies have shown that replacing the oxygen-bearing substituents at C-10 with an amino group, as in this compound, can lead to enhanced activity against both asexual and sexual blood stages of the malaria parasite [, ].
ANone: Yes, researchers have synthesized and evaluated various this compound derivatives to explore structure-activity relationships and optimize its properties [, , , ]. For instance, the synthesis of N-glycosylated derivatives of this compound has shown promising results, with some exhibiting superior antimalarial activity and selectivity compared to this compound itself []. These findings demonstrate the potential for structural optimization of this compound to further improve its therapeutic profile.
ANone: Various strategies have been investigated to enhance this compound's stability, solubility, and bioavailability:
- Self-Microemulsifying Drug Delivery System (SMEDDS): A SMEDDS formulation using Capmul MCM, Kolliphor HS15, propylene glycol, and Polysorb ID 46 demonstrated high drug loading, storage stability, and enhanced oral bioavailability in mice infected with Schistosoma mansoni [, ].
- Lipid Matrix Tablets: Incorporating this compound into lipid matrix tablets, especially in combination with Lumefantrine, has been proposed as a strategy to improve solubility and bioavailability [].
- Pheroid® Drug Delivery System: Although the Pheroid system didn't significantly enhance this compound's in vitro activity, it showed some promise in improving its pharmacokinetic profile in primates [].
- Injectable Pasty Polymer: A slow-release formulation using a poly(sebacic acid–ricinoleic acid) (PSARA) pasty polymer demonstrated sustained release of this compound over several days, enhancing its efficacy in a mouse model of cerebral malaria [].
- Nano- and Microparticles: Encapsulating this compound in biodegradable poly(lactide-co-glycolide) micro- and nanoparticles, prepared via solvent displacement and spray drying methods, has shown promise in achieving controlled release and high drug loading [].
ANone: this compound exhibits favorable pharmacokinetic properties, including dose linearity over a range of doses []. Following a single oral dose of 80 mg in healthy subjects, this compound reached a geometric mean maximum concentration (Cmax) of 140.2 ng/ml with a short elimination half-life (t1/2) of 2.79 hours []. This short half-life is comparable to other artemisinins and makes it suitable for use in artemisinin-based combination therapies (ACTs) [].
ANone: this compound is metabolized into several metabolites, including the active metabolite M1 [, ]. Unlike some other artemisinin derivatives, dihydroartemisinin (DHA) is not a metabolite of this compound []. Its metabolic profile contributes to its overall efficacy and distinguishes it from other artemisinins.
ANone: Yes, research suggests that the route of administration can significantly impact this compound's effectiveness. In a study using a mouse model of cerebral malaria, intranasal administration of an this compound-SMEDDS formulation proved to be the most efficacious route, followed by oral administration. Intraperitoneal injection was the least effective route []. These findings highlight the importance of optimizing the delivery route for this compound to maximize its therapeutic benefit.
ANone: this compound demonstrates potent in vitro activity against P. falciparum, with 3-5 fold higher potency than artesunate [, ]. Its activity remains consistent across different strains, including multidrug-resistant ones [].
ANone: this compound's antimalarial activity has been extensively evaluated in various animal models, including:
- Aotus Monkeys: this compound, in combination with mefloquine or amodiaquine, effectively cured P. falciparum infections in Aotus monkeys [].
- Mice: Studies using a mouse model of cerebral malaria (Plasmodium berghei ANKA) have shown that this compound, particularly in combination with other antimalarial drugs and when formulated for controlled release, can effectively reduce parasitemia, prolong survival, and prevent death [, , ].
ANone: Yes, this compound has undergone Phase I clinical trials in humans. These studies have demonstrated its safety, tolerability, and favorable pharmacokinetic profile []. While further clinical trials are needed to establish its efficacy in treating malaria in humans, the initial results are promising.
ANone: While this compound has shown promising activity against drug-resistant P. falciparum strains in vitro, the potential for resistance development remains a concern, as with all antimalarial drugs. Monitoring for resistance emergence is crucial for its long-term effectiveness.
ANone: Apart from the formulation strategies mentioned earlier, researchers are investigating ways to enhance this compound's delivery to its target, specifically within the parasite. One approach could involve utilizing nanocarriers that specifically target infected red blood cells, improving drug concentration at the site of action and potentially reducing systemic toxicity [].
ANone: Various analytical techniques have been employed to characterize and quantify this compound and its metabolites, including:
- Liquid Chromatography-Tandem Mass Spectrometry (LC/MS-MS): This highly sensitive and specific method has been used to measure plasma concentrations of this compound and its metabolites in pharmacokinetic studies [, ].
- High-Performance Liquid Chromatography (HPLC): HPLC, often coupled with UV detection or mass spectrometry, has been used for the quantification of this compound in various matrices, including plasma, in vitro cultures, and formulations [, , ].
- Enzyme-Linked Immunosorbent Assay (ELISA): ELISA, specifically the histidine-rich protein 2 (HRP2) assay, has been employed to assess the ex vivo activity of this compound against P. falciparum isolates [].
ANone: this compound exhibits poor aqueous solubility, posing a challenge for its formulation and bioavailability [, ]. This limitation has driven the exploration of various drug delivery systems, such as SMEDDS and lipid-based formulations, to enhance its solubility and dissolution rate, ultimately improving its absorption and therapeutic efficacy.
ANone: Research suggests that this compound might interact with efflux transporters, specifically P-glycoprotein (P-gp), which could affect its absorption and bioavailability [, ]. Further investigation is necessary to fully elucidate these interactions and their clinical implications for this compound's use, especially in combination therapies.
ANone: Yes, the ongoing search for new antimalarial treatments is driven by the emergence of drug resistance and the need for more effective therapies. Several alternatives and potential substitutes for this compound are under investigation, including:
- Synthetic Peroxides: OZ277 (Arterolane) and OZ439 are fully synthetic ozonides that have shown promising antimalarial activity and are in clinical trials [].
- Other Artemisinin Derivatives: Researchers continue to explore new artemisinin derivatives with improved properties, such as enhanced activity against resistant parasites, longer half-lives, and reduced toxicity [, , , ].
- Combinations with Redox Active Drugs: Combining this compound or other artemisinin derivatives with redox active drugs like methylene blue is a promising strategy to enhance antimalarial activity, particularly against gametocytes [, ].
- High-throughput Screening Platforms: These platforms allow for rapid screening of large libraries of compounds for antimalarial activity, accelerating drug discovery [].
- In Vitro Culture Systems: Continuous in vitro cultures of P. falciparum are essential for evaluating drug efficacy and investigating parasite biology [].
- Animal Models: Various animal models, such as mice and Aotus monkeys, are crucial for preclinical testing of this compound's efficacy and safety [, , , ].
Disclaimer and Information on In-Vitro Research Products
Please be aware that all articles and product information presented on BenchChem are intended solely for informational purposes. The products available for purchase on BenchChem are specifically designed for in-vitro studies, which are conducted outside of living organisms. In-vitro studies, derived from the Latin term "in glass," involve experiments performed in controlled laboratory settings using cells or tissues. It is important to note that these products are not categorized as medicines or drugs, and they have not received approval from the FDA for the prevention, treatment, or cure of any medical condition, ailment, or disease. We must emphasize that any form of bodily introduction of these products into humans or animals is strictly prohibited by law. It is essential to adhere to these guidelines to ensure compliance with legal and ethical standards in research and experimentation.