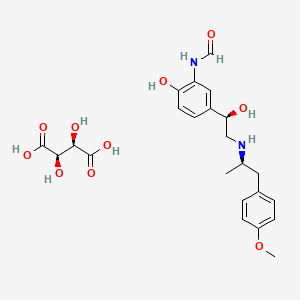
Arformoterol tartrate
Overview
Description
Arformoterol tartrate is a long-acting beta2-adrenergic agonist used primarily as a bronchodilator for the treatment of chronic obstructive pulmonary disease (COPD), including chronic bronchitis and emphysema . It is the (R,R)-enantiomer of formoterol and is marketed under the brand name Brovana . This compound works by relaxing the muscles in the airways to improve breathing .
Preparation Methods
The preparation of arformoterol tartrate involves several synthetic routes. One notable method includes the preparation of arformoterol L-(+)-tartrate via arformoterol D-(-)-tartrate . The process involves the use of specific reagents and conditions to achieve the desired enantiomeric purity. Industrial production methods typically involve the synthesis of arformoterol followed by its conversion to the tartrate salt, ensuring the compound’s stability and efficacy for medical use .
Chemical Reactions Analysis
Arformoterol tartrate undergoes various chemical reactions, including oxidation, reduction, and substitution reactions. Common reagents used in these reactions include oxidizing agents like potassium permanganate and reducing agents such as lithium aluminum hydride . The major products formed from these reactions depend on the specific conditions and reagents used. For instance, oxidation of arformoterol can lead to the formation of corresponding ketones or carboxylic acids .
Scientific Research Applications
Arformoterol tartrate has a wide range of scientific research applications. In medicine, it is used for the long-term maintenance treatment of bronchoconstriction in patients with COPD . In chemistry, it serves as a model compound for studying beta2-adrenergic agonists and their interactions with receptors . In biology, this compound is used to investigate the mechanisms of bronchial smooth muscle relaxation and the inhibition of mediator release from mast cells . Additionally, it has industrial applications in the formulation of inhalation solutions for respiratory therapy .
Mechanism of Action
Arformoterol tartrate exerts its effects by selectively binding to beta2-adrenergic receptors in the bronchial smooth muscle . This binding activates intracellular adenyl cyclase, which catalyzes the conversion of adenosine triphosphate (ATP) to cyclic adenosine monophosphate (cAMP) . Increased levels of cAMP lead to the relaxation of bronchial smooth muscle and inhibition of mediator release from mast cells . The compound’s high selectivity for beta2-receptors minimizes its effects on the cardiovascular system .
Comparison with Similar Compounds
Arformoterol tartrate is often compared with other long-acting beta2-adrenergic agonists such as formoterol, salmeterol, and indacaterol . Unlike racemic formoterol, this compound is the pure (R,R)-enantiomer, which provides greater potency and selectivity . Salmeterol and indacaterol also serve as bronchodilators but differ in their duration of action and receptor selectivity . Arformoterol’s unique enantiomeric purity and high potency make it a valuable option for the treatment of COPD .
Properties
IUPAC Name |
(2R,3R)-2,3-dihydroxybutanedioic acid;N-[2-hydroxy-5-[(1R)-1-hydroxy-2-[[(2R)-1-(4-methoxyphenyl)propan-2-yl]amino]ethyl]phenyl]formamide | |
---|---|---|
Source | PubChem | |
URL | https://pubchem.ncbi.nlm.nih.gov | |
Description | Data deposited in or computed by PubChem | |
InChI |
InChI=1S/C19H24N2O4.C4H6O6/c1-13(9-14-3-6-16(25-2)7-4-14)20-11-19(24)15-5-8-18(23)17(10-15)21-12-22;5-1(3(7)8)2(6)4(9)10/h3-8,10,12-13,19-20,23-24H,9,11H2,1-2H3,(H,21,22);1-2,5-6H,(H,7,8)(H,9,10)/t13-,19+;1-,2-/m11/s1 | |
Source | PubChem | |
URL | https://pubchem.ncbi.nlm.nih.gov | |
Description | Data deposited in or computed by PubChem | |
InChI Key |
FCSXYHUNDAXDRH-OKMNHOJOSA-N | |
Source | PubChem | |
URL | https://pubchem.ncbi.nlm.nih.gov | |
Description | Data deposited in or computed by PubChem | |
Canonical SMILES |
CC(CC1=CC=C(C=C1)OC)NCC(C2=CC(=C(C=C2)O)NC=O)O.C(C(C(=O)O)O)(C(=O)O)O | |
Source | PubChem | |
URL | https://pubchem.ncbi.nlm.nih.gov | |
Description | Data deposited in or computed by PubChem | |
Isomeric SMILES |
C[C@H](CC1=CC=C(C=C1)OC)NC[C@@H](C2=CC(=C(C=C2)O)NC=O)O.[C@@H]([C@H](C(=O)O)O)(C(=O)O)O | |
Source | PubChem | |
URL | https://pubchem.ncbi.nlm.nih.gov | |
Description | Data deposited in or computed by PubChem | |
Molecular Formula |
C23H30N2O10 | |
Source | PubChem | |
URL | https://pubchem.ncbi.nlm.nih.gov | |
Description | Data deposited in or computed by PubChem | |
DSSTOX Substance ID |
DTXSID80173903 | |
Record name | Arformoterol tartrate | |
Source | EPA DSSTox | |
URL | https://comptox.epa.gov/dashboard/DTXSID80173903 | |
Description | DSSTox provides a high quality public chemistry resource for supporting improved predictive toxicology. | |
Molecular Weight |
494.5 g/mol | |
Source | PubChem | |
URL | https://pubchem.ncbi.nlm.nih.gov | |
Description | Data deposited in or computed by PubChem | |
CAS No. |
200815-49-2 | |
Record name | Arformoterol Tartrate [USAN] | |
Source | ChemIDplus | |
URL | https://pubchem.ncbi.nlm.nih.gov/substance/?source=chemidplus&sourceid=0200815492 | |
Description | ChemIDplus is a free, web search system that provides access to the structure and nomenclature authority files used for the identification of chemical substances cited in National Library of Medicine (NLM) databases, including the TOXNET system. | |
Record name | Arformoterol tartrate | |
Source | EPA DSSTox | |
URL | https://comptox.epa.gov/dashboard/DTXSID80173903 | |
Description | DSSTox provides a high quality public chemistry resource for supporting improved predictive toxicology. | |
Record name | (R, R)-(-)-N-[2-hydroxy-5-[1-hydroxy-2-[[2-(4-methoxyphenyl)-1-methylethyl] amino]ethyl] phenylformamide (2R, 3R)-dihydroxy butanedioate, 1:1 salt | |
Source | European Chemicals Agency (ECHA) | |
URL | https://echa.europa.eu/information-on-chemicals | |
Description | The European Chemicals Agency (ECHA) is an agency of the European Union which is the driving force among regulatory authorities in implementing the EU's groundbreaking chemicals legislation for the benefit of human health and the environment as well as for innovation and competitiveness. | |
Explanation | Use of the information, documents and data from the ECHA website is subject to the terms and conditions of this Legal Notice, and subject to other binding limitations provided for under applicable law, the information, documents and data made available on the ECHA website may be reproduced, distributed and/or used, totally or in part, for non-commercial purposes provided that ECHA is acknowledged as the source: "Source: European Chemicals Agency, http://echa.europa.eu/". Such acknowledgement must be included in each copy of the material. ECHA permits and encourages organisations and individuals to create links to the ECHA website under the following cumulative conditions: Links can only be made to webpages that provide a link to the Legal Notice page. | |
Record name | Arformoterol Tartrate | |
Source | FDA Global Substance Registration System (GSRS) | |
URL | https://gsrs.ncats.nih.gov/ginas/app/beta/substances/5P8VJ2I235 | |
Description | The FDA Global Substance Registration System (GSRS) enables the efficient and accurate exchange of information on what substances are in regulated products. Instead of relying on names, which vary across regulatory domains, countries, and regions, the GSRS knowledge base makes it possible for substances to be defined by standardized, scientific descriptions. | |
Explanation | Unless otherwise noted, the contents of the FDA website (www.fda.gov), both text and graphics, are not copyrighted. They are in the public domain and may be republished, reprinted and otherwise used freely by anyone without the need to obtain permission from FDA. Credit to the U.S. Food and Drug Administration as the source is appreciated but not required. | |
Retrosynthesis Analysis
AI-Powered Synthesis Planning: Our tool employs the Template_relevance Pistachio, Template_relevance Bkms_metabolic, Template_relevance Pistachio_ringbreaker, Template_relevance Reaxys, Template_relevance Reaxys_biocatalysis model, leveraging a vast database of chemical reactions to predict feasible synthetic routes.
One-Step Synthesis Focus: Specifically designed for one-step synthesis, it provides concise and direct routes for your target compounds, streamlining the synthesis process.
Accurate Predictions: Utilizing the extensive PISTACHIO, BKMS_METABOLIC, PISTACHIO_RINGBREAKER, REAXYS, REAXYS_BIOCATALYSIS database, our tool offers high-accuracy predictions, reflecting the latest in chemical research and data.
Strategy Settings
Precursor scoring | Relevance Heuristic |
---|---|
Min. plausibility | 0.01 |
Model | Template_relevance |
Template Set | Pistachio/Bkms_metabolic/Pistachio_ringbreaker/Reaxys/Reaxys_biocatalysis |
Top-N result to add to graph | 6 |
Feasible Synthetic Routes
Disclaimer and Information on In-Vitro Research Products
Please be aware that all articles and product information presented on BenchChem are intended solely for informational purposes. The products available for purchase on BenchChem are specifically designed for in-vitro studies, which are conducted outside of living organisms. In-vitro studies, derived from the Latin term "in glass," involve experiments performed in controlled laboratory settings using cells or tissues. It is important to note that these products are not categorized as medicines or drugs, and they have not received approval from the FDA for the prevention, treatment, or cure of any medical condition, ailment, or disease. We must emphasize that any form of bodily introduction of these products into humans or animals is strictly prohibited by law. It is essential to adhere to these guidelines to ensure compliance with legal and ethical standards in research and experimentation.