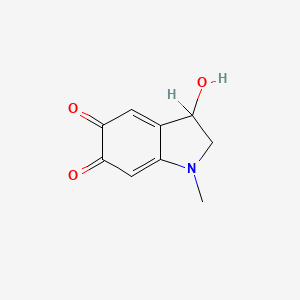
Adrenochrome
Overview
Description
Mechanism of Action
Target of Action
Adrenochrome is an oxidation product of adrenaline . It is part of the leukocyte response used to kill bacteria . .
Mode of Action
The oxidation reaction that converts adrenaline into this compound occurs both in vivo and in vitro . Silver oxide (Ag 2 O) was among the first reagents employed for this, but a variety of other oxidizing agents have been used successfully .
Biochemical Pathways
This compound is produced in the body by the oxidation of the hormone adrenaline . The oxidation reaction that converts adrenaline into this compound occurs both in vivo and in vitro . Further oxidation of the compound causes it to polymerize into brown or black melanin compounds .
Pharmacokinetics
This compound is an unstable molecule . Its monosemicarbazone (carbazochrome) is stable and is said to promote blood clotting and can be used to treat hemorrhaging . .
Result of Action
This compound is suggested to exhibit psychotomimetic effects . Several small-scale studies conducted in the 1950s and 1960s reported that this compound triggered psychotic reactions such as thought disorder and derealization . These findings have been contested, and the theory that this compound causes schizophrenia has been debunked .
Action Environment
The environment can influence the action of this compound. For instance, the oxidation reaction that converts adrenaline into this compound can occur both inside the body (in vivo) and outside the body (in vitro) . .
Biochemical Analysis
Biochemical Properties
Adrenochrome is formed by the oxidation of adrenaline . The two major catabolites on this pathway are this compound and adrenolutin . Over the concentration range 0.3-10 microM, more than 80% of the adrenaline oxidation occurred via the this compound pathway .
Cellular Effects
This compound has been reported to trigger psychotic reactions such as thought disorder and derealization .
Molecular Mechanism
The oxidation reaction that converts adrenaline into this compound occurs both in vivo and in vitro . Silver oxide (Ag2O) was among the first reagents employed for this, but a variety of other oxidizing agents have been used successfully .
Temporal Effects in Laboratory Settings
In laboratory settings, this compound has been observed to trigger effects such as thought disorder and derealization
Metabolic Pathways
This compound is a part of the this compound pathway, which is a potential catabolic route for adrenaline metabolism . The two major catabolites on this pathway are this compound and adrenolutin .
Preparation Methods
Adrenochrome can be synthesized through the oxidation of adrenaline. One common method involves using silver oxide (Ag₂O) as the oxidizing agent . Another method employs persulfates in an aqueous medium at a pH range of 4 to 8, often in the presence of water-soluble salts of bismuth . Industrial production methods typically focus on optimizing yield and purity, with considerations for cost-effectiveness and environmental impact .
Chemical Reactions Analysis
Adrenochrome undergoes several types of chemical reactions:
Oxidation: this compound is formed by the oxidation of adrenaline.
Reduction: this compound can be reduced back to adrenaline under specific conditions.
Polymerization: In solution, this compound can polymerize, especially under alkaline conditions, forming various colored compounds.
Common reagents used in these reactions include silver oxide, persulfates, and other oxidizing agents . The major products formed from these reactions are this compound itself, adrenolutin, and melanin compounds .
Scientific Research Applications
Adrenochrome has been the subject of various scientific studies, particularly in the mid-20th century. Some of its applications include:
Psychiatric Research: This compound was once hypothesized to be linked to schizophrenia due to its structural similarity to mescaline.
Hemostatic Agent:
Biological Studies: This compound is used in studies related to oxidative stress and the activity of antioxidants.
Comparison with Similar Compounds
Adrenochrome is similar to several other compounds, including:
Adrenaline (Epinephrine): The precursor to this compound, adrenaline is a hormone and neurotransmitter involved in the body’s fight-or-flight response.
Carbazochrome: A stabilized form of this compound used as a hemostatic agent.
This compound’s uniqueness lies in its vivid coloration and its historical significance in psychiatric research, despite its limited practical applications today .
Properties
IUPAC Name |
3-hydroxy-1-methyl-2,3-dihydroindole-5,6-dione | |
---|---|---|
Source | PubChem | |
URL | https://pubchem.ncbi.nlm.nih.gov | |
Description | Data deposited in or computed by PubChem | |
InChI |
InChI=1S/C9H9NO3/c1-10-4-9(13)5-2-7(11)8(12)3-6(5)10/h2-3,9,13H,4H2,1H3 | |
Source | PubChem | |
URL | https://pubchem.ncbi.nlm.nih.gov | |
Description | Data deposited in or computed by PubChem | |
InChI Key |
RPHLQSHHTJORHI-UHFFFAOYSA-N | |
Source | PubChem | |
URL | https://pubchem.ncbi.nlm.nih.gov | |
Description | Data deposited in or computed by PubChem | |
Canonical SMILES |
CN1CC(C2=CC(=O)C(=O)C=C21)O | |
Source | PubChem | |
URL | https://pubchem.ncbi.nlm.nih.gov | |
Description | Data deposited in or computed by PubChem | |
Molecular Formula |
C9H9NO3 | |
Source | PubChem | |
URL | https://pubchem.ncbi.nlm.nih.gov | |
Description | Data deposited in or computed by PubChem | |
DSSTOX Substance ID |
DTXSID00871530 | |
Record name | 3-Hydroxy-1-methyl-2,3-dihydro-1H-indole-5,6-dione | |
Source | EPA DSSTox | |
URL | https://comptox.epa.gov/dashboard/DTXSID00871530 | |
Description | DSSTox provides a high quality public chemistry resource for supporting improved predictive toxicology. | |
Molecular Weight |
179.17 g/mol | |
Source | PubChem | |
URL | https://pubchem.ncbi.nlm.nih.gov | |
Description | Data deposited in or computed by PubChem | |
CAS No. |
54-06-8, 7506-92-5 | |
Record name | Adrenochrome | |
Source | CAS Common Chemistry | |
URL | https://commonchemistry.cas.org/detail?cas_rn=54-06-8 | |
Description | CAS Common Chemistry is an open community resource for accessing chemical information. Nearly 500,000 chemical substances from CAS REGISTRY cover areas of community interest, including common and frequently regulated chemicals, and those relevant to high school and undergraduate chemistry classes. This chemical information, curated by our expert scientists, is provided in alignment with our mission as a division of the American Chemical Society. | |
Explanation | The data from CAS Common Chemistry is provided under a CC-BY-NC 4.0 license, unless otherwise stated. | |
Record name | Adrenochrome | |
Source | ChemIDplus | |
URL | https://pubchem.ncbi.nlm.nih.gov/substance/?source=chemidplus&sourceid=0000054068 | |
Description | ChemIDplus is a free, web search system that provides access to the structure and nomenclature authority files used for the identification of chemical substances cited in National Library of Medicine (NLM) databases, including the TOXNET system. | |
Record name | 1-Adrenochrome | |
Source | DTP/NCI | |
URL | https://dtp.cancer.gov/dtpstandard/servlet/dwindex?searchtype=NSC&outputformat=html&searchlist=407840 | |
Description | The NCI Development Therapeutics Program (DTP) provides services and resources to the academic and private-sector research communities worldwide to facilitate the discovery and development of new cancer therapeutic agents. | |
Explanation | Unless otherwise indicated, all text within NCI products is free of copyright and may be reused without our permission. Credit the National Cancer Institute as the source. | |
Record name | 3-Hydroxy-1-methyl-2,3-dihydro-1H-indole-5,6-dione | |
Source | EPA DSSTox | |
URL | https://comptox.epa.gov/dashboard/DTXSID00871530 | |
Description | DSSTox provides a high quality public chemistry resource for supporting improved predictive toxicology. | |
Record name | 3-hydroxy-1-methylindoline-5,6-dione | |
Source | European Chemicals Agency (ECHA) | |
URL | https://echa.europa.eu/substance-information/-/substanceinfo/100.000.176 | |
Description | The European Chemicals Agency (ECHA) is an agency of the European Union which is the driving force among regulatory authorities in implementing the EU's groundbreaking chemicals legislation for the benefit of human health and the environment as well as for innovation and competitiveness. | |
Explanation | Use of the information, documents and data from the ECHA website is subject to the terms and conditions of this Legal Notice, and subject to other binding limitations provided for under applicable law, the information, documents and data made available on the ECHA website may be reproduced, distributed and/or used, totally or in part, for non-commercial purposes provided that ECHA is acknowledged as the source: "Source: European Chemicals Agency, http://echa.europa.eu/". Such acknowledgement must be included in each copy of the material. ECHA permits and encourages organisations and individuals to create links to the ECHA website under the following cumulative conditions: Links can only be made to webpages that provide a link to the Legal Notice page. | |
Record name | ADRENOCHROME | |
Source | FDA Global Substance Registration System (GSRS) | |
URL | https://gsrs.ncats.nih.gov/ginas/app/beta/substances/70G54NQL71 | |
Description | The FDA Global Substance Registration System (GSRS) enables the efficient and accurate exchange of information on what substances are in regulated products. Instead of relying on names, which vary across regulatory domains, countries, and regions, the GSRS knowledge base makes it possible for substances to be defined by standardized, scientific descriptions. | |
Explanation | Unless otherwise noted, the contents of the FDA website (www.fda.gov), both text and graphics, are not copyrighted. They are in the public domain and may be republished, reprinted and otherwise used freely by anyone without the need to obtain permission from FDA. Credit to the U.S. Food and Drug Administration as the source is appreciated but not required. | |
Retrosynthesis Analysis
AI-Powered Synthesis Planning: Our tool employs the Template_relevance Pistachio, Template_relevance Bkms_metabolic, Template_relevance Pistachio_ringbreaker, Template_relevance Reaxys, Template_relevance Reaxys_biocatalysis model, leveraging a vast database of chemical reactions to predict feasible synthetic routes.
One-Step Synthesis Focus: Specifically designed for one-step synthesis, it provides concise and direct routes for your target compounds, streamlining the synthesis process.
Accurate Predictions: Utilizing the extensive PISTACHIO, BKMS_METABOLIC, PISTACHIO_RINGBREAKER, REAXYS, REAXYS_BIOCATALYSIS database, our tool offers high-accuracy predictions, reflecting the latest in chemical research and data.
Strategy Settings
Precursor scoring | Relevance Heuristic |
---|---|
Min. plausibility | 0.01 |
Model | Template_relevance |
Template Set | Pistachio/Bkms_metabolic/Pistachio_ringbreaker/Reaxys/Reaxys_biocatalysis |
Top-N result to add to graph | 6 |
Feasible Synthetic Routes
Q1: How does adrenochrome interact with biological systems and what are the downstream effects?
A1: this compound interacts with various cellular components, including mitochondria and the sarcoplasmic reticulum, influencing calcium handling and energy production within cells. [, ] Studies have shown that this compound can inhibit calcium binding, calcium uptake, and calcium-stimulated magnesium-dependent adenosine triphosphatase (ATPase) activities in rat heart microsomes. [] This interference with calcium regulation is thought to contribute to its cardiotoxic effects.
Q2: What is the molecular formula, weight, and spectroscopic data of this compound?
A2: this compound has the molecular formula C9H9NO3 and a molecular weight of 179.17 g/mol. Spectroscopically, it is characterized by distinct absorption peaks at approximately 220 nm, 302 nm, and 485 nm. []
Q3: What enzymes are involved in the metabolism of this compound?
A3: Research suggests that both NADPH-cytochrome P450 reductase and DT-diaphorase play a role in this compound metabolism. These enzymes catalyze the one- and two-electron reduction of this compound to its o-semiquinone and o-hydroquinone forms, respectively. [] The enzyme glutathione S-transferase is also believed to be involved in this compound detoxification. []
Q4: How does the presence of reducing agents influence this compound's effects on the heart?
A4: The addition of reducing agents like ascorbic acid or cysteine to this compound-perfused hearts was found to exacerbate ultrastructural damage compared to this compound alone. [] This suggests that the reduction of this compound to other catecholamine oxidation products may contribute to its cardiotoxicity, potentially through the formation of free radicals or interactions with sulfhydryl groups. []
Q5: What is the role of oxygen free radicals in this compound-induced cardiotoxicity?
A5: While this compound itself may not directly generate superoxide anion radicals, its formation and subsequent metabolism involve redox reactions that can indirectly contribute to oxidative stress within cells. [, ] This oxidative stress is thought to be a significant contributor to the cardiotoxic effects observed in various studies.
Q6: How do structural modifications of this compound affect its biological activity?
A6: While this compound itself exhibits biological activity, research indicates that some of its effects might stem from contaminants in commercially available preparations. [] Structural modifications, such as the formation of this compound semicarbazone, are known to alter its pharmacological properties, including its stability and effects on platelet aggregation. [, ] Further research is needed to fully elucidate the structure-activity relationships of this compound and its derivatives.
Q7: How stable is this compound under various conditions, and what strategies can be employed to enhance its stability?
A7: this compound is known to be unstable in its pure form, readily undergoing oxidation and polymerization. [, ] The development of stable formulations, such as the this compound monosemicarbazone complex, has been crucial for studying its biological effects. [] Strategies to improve stability often involve complexation with stabilizing agents or the development of specific delivery systems.
Q8: What are the known toxicological effects of this compound, and what safety measures should be considered?
A8: Research on this compound toxicity has highlighted its potential to induce cardiac contractile failure and myocardial necrosis, particularly at high concentrations. [, ] These effects are thought to be mediated through its interaction with cellular calcium handling, mitochondrial function, and the generation of reactive oxygen species. [, ] Further research is necessary to fully elucidate the toxicological profile of this compound and establish safe exposure limits.
Q9: What analytical techniques are commonly employed to detect and quantify this compound?
A10: High-performance liquid chromatography (HPLC) coupled with photodiode array detection has been successfully utilized to analyze this compound and related compounds. [] This method allows for the separation and quantification of this compound in complex biological matrices. Additionally, UV-Vis spectrophotometry can be used to track this compound formation and degradation based on its characteristic absorption peaks. []
Q10: What are some key historical milestones in this compound research?
A11: Early research in the 1950s explored the potential role of this compound in schizophrenia, although those theories remain largely unsubstantiated. [, ] Subsequent research focused on understanding its formation, metabolism, and biological effects, particularly in the context of cardiac function and oxidative stress. [, ] More recent studies have investigated its interactions with specific enzymes, including DT-diaphorase and NADPH-cytochrome P450 reductase. []
Disclaimer and Information on In-Vitro Research Products
Please be aware that all articles and product information presented on BenchChem are intended solely for informational purposes. The products available for purchase on BenchChem are specifically designed for in-vitro studies, which are conducted outside of living organisms. In-vitro studies, derived from the Latin term "in glass," involve experiments performed in controlled laboratory settings using cells or tissues. It is important to note that these products are not categorized as medicines or drugs, and they have not received approval from the FDA for the prevention, treatment, or cure of any medical condition, ailment, or disease. We must emphasize that any form of bodily introduction of these products into humans or animals is strictly prohibited by law. It is essential to adhere to these guidelines to ensure compliance with legal and ethical standards in research and experimentation.