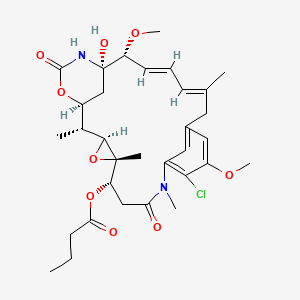
Maytansinol butyrate
Overview
Description
Mechanism of Action
Target of Action
Maytansinol butyrate, also known as ansamitocin P 3’, is an antibody-drug conjugate cytotoxin . It primarily targets antigen-positive cells for treating cancer . The compound’s primary target is the lysosomal membrane protein SLC46A3 . This protein is responsible for transporting noncleavable antibody maytansine conjugates from the lysosome to the cytoplasm .
Mode of Action
This compound interacts with its targets by inhibiting the assembly of microtubules . It binds to tubulin at the rhizoxin binding site . The monoclonal antibody in the compound binds specifically to a structure (usually a protein) occurring in a tumor, thus directing this compound into this tumor .
Biochemical Pathways
The compound affects the acetyl-coenzyme A (CoA) pathway and the lysine pathway . These pathways are associated with the production of butyrate . The acetyl-CoA pathway is the most prevalent, followed by the lysine pathway .
Pharmacokinetics
This compound, as part of an antibody-drug conjugate (ADC), undergoes biotransformations in circulation . After internalization, ADCs with noncleavable linkers are catabolized to amino acid-linker-warheads within the lysosome, which then enter the cytoplasm .
Result of Action
The result of the action of this compound is the inhibition of microtubule assembly . This leads to the destabilization of microtubules, which are crucial for cell division and intracellular transport . As a result, the compound exhibits antitumor activity .
Action Environment
The action, efficacy, and stability of this compound can be influenced by various environmental factors. For instance, the reaction conditions used for the acylation reaction of maytansinol can significantly influence the formation of diverse products . Additionally, the compound’s action can be affected by the presence of other molecules in the environment, such as other drugs or substances .
Biochemical Analysis
Biochemical Properties
Maytansinol butyrate plays a significant role in biochemical reactions, particularly in its interaction with microtubules. It binds to tubulin, the protein subunit of microtubules, and inhibits their polymerization. This interaction disrupts the microtubule dynamics necessary for cell division, leading to cell cycle arrest and apoptosis . The compound also interacts with various enzymes and proteins involved in cell signaling pathways, such as kinases and phosphatases, further influencing cellular functions .
Cellular Effects
This compound exerts profound effects on various types of cells and cellular processes. It primarily targets rapidly dividing cancer cells, causing mitotic arrest and subsequent cell death. The compound influences cell signaling pathways, including those involved in cell cycle regulation and apoptosis . Additionally, this compound affects gene expression by modulating the activity of transcription factors and histone deacetylases, leading to changes in cellular metabolism and function .
Molecular Mechanism
The molecular mechanism of action of this compound involves its binding to tubulin, which prevents the polymerization of microtubules. This inhibition disrupts the formation of the mitotic spindle, essential for chromosome segregation during cell division . The compound also induces the formation of tubulin aggregates, further impairing microtubule function . Additionally, this compound modulates gene expression by inhibiting histone deacetylases, leading to changes in chromatin structure and transcriptional activity .
Temporal Effects in Laboratory Settings
In laboratory settings, the effects of this compound change over time. The compound is relatively stable under standard storage conditions but can degrade under extreme conditions such as high temperatures or prolonged exposure to light . Long-term studies have shown that this compound maintains its cytotoxic effects on cancer cells over extended periods, although its potency may decrease slightly due to degradation . In vitro and in vivo studies have demonstrated that the compound can induce sustained cell cycle arrest and apoptosis in cancer cells .
Dosage Effects in Animal Models
The effects of this compound vary with different dosages in animal models. At low doses, the compound effectively inhibits tumor growth with minimal toxicity . At higher doses, this compound can cause adverse effects such as weight loss, organ toxicity, and immunosuppression . Threshold effects have been observed, where a certain dosage is required to achieve significant antitumor activity without causing severe side effects .
Metabolic Pathways
This compound is involved in several metabolic pathways, primarily those related to its biotransformation and elimination. The compound is metabolized by liver enzymes, including cytochrome P450 enzymes, which convert it into various metabolites . These metabolites can be further conjugated with glucuronic acid or sulfate, facilitating their excretion . This compound also affects metabolic flux by altering the levels of key metabolites involved in cellular energy production and biosynthesis .
Transport and Distribution
Within cells and tissues, this compound is transported and distributed through various mechanisms. The compound is taken up by cells via passive diffusion and active transport mediated by specific transporters such as monocarboxylate transporter 1 (MCT-1) . Once inside the cell, this compound can bind to intracellular proteins and accumulate in specific cellular compartments . Its distribution within tissues is influenced by factors such as blood flow, tissue permeability, and binding to plasma proteins .
Subcellular Localization
This compound exhibits specific subcellular localization, which is crucial for its activity and function. The compound primarily localizes to the cytoplasm, where it interacts with microtubules and other cytoskeletal components . It can also be found in the nucleus, where it modulates gene expression by inhibiting histone deacetylases . The subcellular localization of this compound is influenced by targeting signals and post-translational modifications that direct it to specific compartments or organelles .
Preparation Methods
Synthetic Routes and Reaction Conditions
Maytansinol butyrate can be synthesized through the acylation of maytansinol. The acylation reaction typically involves the use of acylating agents such as butyric anhydride or butyryl chloride in the presence of a base like triethylamine . The reaction is carried out in a solvent such as dichloromethane at room temperature . The reaction conditions, including the choice of acylating agent, base, and solvent, can significantly influence the yield and purity of the final product .
Industrial Production Methods
Industrial production of this compound involves scaling up the synthetic routes while optimizing reaction conditions to maximize yield and minimize impurities . The process typically includes steps such as reduction of C3-esters of maytansinol using aluminum-based hydride reducing agents, followed by acylation . The use of efficient coupling agents and solvents, along with controlled reaction times and temperatures, is crucial for industrial-scale production .
Chemical Reactions Analysis
Types of Reactions
Maytansinol butyrate undergoes various chemical reactions, including:
Oxidation: this compound can be oxidized to form corresponding ketones or aldehydes.
Reduction: Reduction reactions can convert this compound back to maytansinol.
Substitution: Nucleophilic substitution reactions can introduce different functional groups at specific positions on the molecule.
Common Reagents and Conditions
Oxidation: Common oxidizing agents include potassium permanganate and chromium trioxide.
Reduction: Aluminum-based hydride reducing agents such as lithium aluminum hydride are commonly used.
Substitution: Reagents like propargyl bromide in the presence of cesium carbonate and potassium iodide can be used for alkylation reactions.
Major Products Formed
The major products formed from these reactions include various derivatives of maytansinol, such as esters, ketones, and aldehydes .
Scientific Research Applications
Maytansinol butyrate has a wide range of scientific research applications:
Chemistry: It is used as a precursor for the synthesis of various maytansinoid derivatives.
Biology: This compound is studied for its interactions with tubulin and its effects on microtubule assembly.
Industry: The compound is used in the production of cytotoxic agents for research and therapeutic purposes.
Comparison with Similar Compounds
Maytansinol butyrate is part of the maytansinoid family, which includes compounds such as maytansine, ansamitocin P-3, and ansamitocin P-4 . These compounds share a similar mechanism of action but differ in their chemical structures and cytotoxicity levels . For example, maytansine and ansamitocin P-3 are highly cytotoxic, while maytansinol itself lacks significant cytotoxic activity . The unique structural features of this compound, such as the butyrate ester group, contribute to its distinct biological activity and potential therapeutic applications .
Properties
IUPAC Name |
(11-chloro-21-hydroxy-12,20-dimethoxy-2,5,9,16-tetramethyl-8,23-dioxo-4,24-dioxa-9,22-diazatetracyclo[19.3.1.110,14.03,5]hexacosa-10,12,14(26),16,18-pentaen-6-yl) butanoate | |
---|---|---|
Details | Computed by Lexichem TK 2.7.0 (PubChem release 2021.05.07) | |
Source | PubChem | |
URL | https://pubchem.ncbi.nlm.nih.gov | |
Description | Data deposited in or computed by PubChem | |
InChI |
InChI=1S/C32H43ClN2O9/c1-8-10-27(37)43-25-16-26(36)35(5)21-14-20(15-22(40-6)28(21)33)13-18(2)11-9-12-24(41-7)32(39)17-23(42-30(38)34-32)19(3)29-31(25,4)44-29/h9,11-12,14-15,19,23-25,29,39H,8,10,13,16-17H2,1-7H3,(H,34,38) | |
Details | Computed by InChI 1.0.6 (PubChem release 2021.05.07) | |
Source | PubChem | |
URL | https://pubchem.ncbi.nlm.nih.gov | |
Description | Data deposited in or computed by PubChem | |
InChI Key |
WLKHTIAFMSHJLG-UHFFFAOYSA-N | |
Details | Computed by InChI 1.0.6 (PubChem release 2021.05.07) | |
Source | PubChem | |
URL | https://pubchem.ncbi.nlm.nih.gov | |
Description | Data deposited in or computed by PubChem | |
Canonical SMILES |
CCCC(=O)OC1CC(=O)N(C2=C(C(=CC(=C2)CC(=CC=CC(C3(CC(C(C4C1(O4)C)C)OC(=O)N3)O)OC)C)OC)Cl)C | |
Details | Computed by OEChem 2.3.0 (PubChem release 2021.05.07) | |
Source | PubChem | |
URL | https://pubchem.ncbi.nlm.nih.gov | |
Description | Data deposited in or computed by PubChem | |
Molecular Formula |
C32H43ClN2O9 | |
Details | Computed by PubChem 2.1 (PubChem release 2021.05.07) | |
Source | PubChem | |
URL | https://pubchem.ncbi.nlm.nih.gov | |
Description | Data deposited in or computed by PubChem | |
Molecular Weight |
635.1 g/mol | |
Details | Computed by PubChem 2.1 (PubChem release 2021.05.07) | |
Source | PubChem | |
URL | https://pubchem.ncbi.nlm.nih.gov | |
Description | Data deposited in or computed by PubChem | |
CAS No. |
66547-09-9 | |
Record name | 2'-De(acetylmethylamino)-2'-methylmaytansine | |
Source | European Chemicals Agency (ECHA) | |
URL | https://echa.europa.eu/information-on-chemicals | |
Description | The European Chemicals Agency (ECHA) is an agency of the European Union which is the driving force among regulatory authorities in implementing the EU's groundbreaking chemicals legislation for the benefit of human health and the environment as well as for innovation and competitiveness. | |
Explanation | Use of the information, documents and data from the ECHA website is subject to the terms and conditions of this Legal Notice, and subject to other binding limitations provided for under applicable law, the information, documents and data made available on the ECHA website may be reproduced, distributed and/or used, totally or in part, for non-commercial purposes provided that ECHA is acknowledged as the source: "Source: European Chemicals Agency, http://echa.europa.eu/". Such acknowledgement must be included in each copy of the material. ECHA permits and encourages organisations and individuals to create links to the ECHA website under the following cumulative conditions: Links can only be made to webpages that provide a link to the Legal Notice page. | |
Retrosynthesis Analysis
AI-Powered Synthesis Planning: Our tool employs the Template_relevance Pistachio, Template_relevance Bkms_metabolic, Template_relevance Pistachio_ringbreaker, Template_relevance Reaxys, Template_relevance Reaxys_biocatalysis model, leveraging a vast database of chemical reactions to predict feasible synthetic routes.
One-Step Synthesis Focus: Specifically designed for one-step synthesis, it provides concise and direct routes for your target compounds, streamlining the synthesis process.
Accurate Predictions: Utilizing the extensive PISTACHIO, BKMS_METABOLIC, PISTACHIO_RINGBREAKER, REAXYS, REAXYS_BIOCATALYSIS database, our tool offers high-accuracy predictions, reflecting the latest in chemical research and data.
Strategy Settings
Precursor scoring | Relevance Heuristic |
---|---|
Min. plausibility | 0.01 |
Model | Template_relevance |
Template Set | Pistachio/Bkms_metabolic/Pistachio_ringbreaker/Reaxys/Reaxys_biocatalysis |
Top-N result to add to graph | 6 |
Feasible Synthetic Routes
Q1: What is the primary molecular target of Maytansinol butyrate and how does it exert its antitumor effects?
A1: this compound primarily targets tubulin, a protein crucial for microtubule formation. [] Microtubules are essential for various cellular processes, including cell division (mitosis). By binding to tubulin at a site distinct from other antimitotic agents like Vinblastine, this compound disrupts microtubule assembly and dynamics, ultimately leading to mitotic arrest and cell death. [, ]
Q2: What is the molecular formula and weight of this compound?
A2: While the provided abstracts don't explicitly state the molecular formula and weight of this compound, they do mention its chemical modifications and derivatives. For example, the synthesis of 3-epimaytansinoids from this compound is described, suggesting a complex structure with multiple modifiable sites. [] To obtain the precise molecular formula and weight, consult chemical databases or the original research articles describing its isolation and characterization.
Q3: How do structural modifications of this compound impact its activity?
A4: Research indicates that even minor structural changes in this compound can significantly affect its biological activity. For example, hydroxylation at the C15, C26, or acyl moiety at the C3 position significantly reduces antitubulin activity, while demethylation at the -NCH3 group on C18 has a milder effect. [] This highlights the importance of the specific stereochemistry and functional groups present in the this compound molecule for its interaction with tubulin and its antitumor effects.
Q4: Have any analogs of this compound been synthesized and what are their characteristics?
A5: Yes, several semi-synthetic this compound analogs, particularly those with variations in the acyl group at the C3 position, have been synthesized and evaluated. [] Some analogs with straight-chain aliphatic acyl, cycloalkanecarbonyl, or phenylacetyl groups at C3, and those with 2-(N-acetyl-N-methyl)aminohexanoyl or (2-(N-acetyl-N-methyl)aminophenylpropionyl groups showed potent antitumor activity comparable to this compound. Notably, a phenylglycinate analog demonstrated a broader effective dose range against B16 melanoma and P388 leukemia in mice than this compound itself. []
Q5: Are there any natural compounds that share structural similarities with this compound and exhibit similar biological activity?
A6: Yes, Rhizoxin, a macrolide antibiotic, shares some structural similarities with this compound and also exhibits potent antitumor activity by inhibiting tubulin polymerization. [, , ] Interestingly, studies with Rhizoxin-resistant mutants of Aspergillus nidulans, which have a single amino acid alteration in their β-tubulin gene, show cross-resistance to this compound, suggesting that these compounds share a similar binding site on tubulin. [, ]
Q6: What is the origin of the "glycolate" unit incorporated into the structure of this compound during its biosynthesis?
A7: Research using isotope-labeled precursors indicates that the "glycolate" unit in this compound originates from 1,3-bisphosphoglycerate, not from hydroxypyruvate or TCA cycle intermediates. [] The C-1 of 1,3-bisphosphoglycerate becomes the thioester carbonyl group (and subsequently C-1 of the "glycolate" unit), while its C-3 is lost during Claisen condensation on the polyketide synthase (PKS) involved in its biosynthesis. []
Q7: What are the potential applications of this compound in medicine?
A9: Given its potent antitumor activity, this compound holds significant promise as a lead compound for developing novel anticancer therapeutics. [, , , ] Its ability to target tubulin and disrupt microtubule dynamics makes it an attractive candidate for targeting rapidly dividing cancer cells. []
Disclaimer and Information on In-Vitro Research Products
Please be aware that all articles and product information presented on BenchChem are intended solely for informational purposes. The products available for purchase on BenchChem are specifically designed for in-vitro studies, which are conducted outside of living organisms. In-vitro studies, derived from the Latin term "in glass," involve experiments performed in controlled laboratory settings using cells or tissues. It is important to note that these products are not categorized as medicines or drugs, and they have not received approval from the FDA for the prevention, treatment, or cure of any medical condition, ailment, or disease. We must emphasize that any form of bodily introduction of these products into humans or animals is strictly prohibited by law. It is essential to adhere to these guidelines to ensure compliance with legal and ethical standards in research and experimentation.