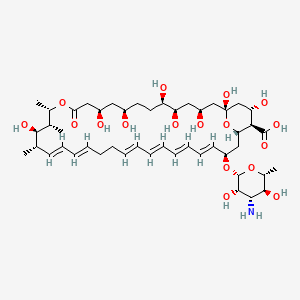
Amphotericin A
Overview
Description
. It is a derivative of Amphotericin B and is known for its potent antifungal properties.
Mechanism of Action
Target of Action
Amphotericin A, like its counterpart Amphotericin B, primarily targets ergosterol , a sterol present in the cell membrane of susceptible fungi . Ergosterol plays a crucial role in maintaining the integrity and fluidity of the fungal cell membrane .
Mode of Action
This compound interacts with its target, ergosterol, by binding to it . This binding forms a transmembrane channel, leading to a change in the membrane’s permeability . The formation of these channels allows for the leakage of intracellular components, disrupting the normal functioning of the cell .
Biochemical Pathways
The primary biochemical pathway affected by this compound is the ergosterol synthesis pathway . By binding to ergosterol, this compound disrupts this pathway, leading to an accumulation of sterol intermediates . This disruption affects the cell’s ability to maintain its membrane integrity, leading to cell death .
Pharmacokinetics
Amphotericin B is known for its long terminal half-life and retention in tissues . These properties suggest that this compound may also have a long half-life and high tissue retention, influencing its bioavailability.
Result of Action
The primary result of this compound’s action is the death of the fungal cell . By disrupting the cell membrane’s integrity through its interaction with ergosterol, this compound causes the cell’s contents to leak out, leading to cell death .
Action Environment
The action of this compound can be influenced by various environmental factors. For instance, the growth phase of fungi and the presence of oxygen can affect the biological effect of this compound . Understanding these environmental influences can help optimize the use of this compound in treating fungal infections.
Biochemical Analysis
Biochemical Properties
Amphotericin A exerts its antifungal effect by disruption of fungal cell wall synthesis due to its ability to bind to sterols, primarily ergosterol . This leads to the formation of pores that allow leakage of cellular components . This affinity may also account for its toxic effects against select mammalian cells .
Cellular Effects
This compound binds preferentially to the cell walls of mature cells but also to the plasma membranes of the daughter cells at the budding stage . It interferes with the formation of functional cell walls of such young cells . It also forms this compound-rich extramembranous structures and penetrates into the cells .
Molecular Mechanism
This compound works by binding to sterols (ergosterol) in the cell membrane of susceptible fungi . This creates a transmembrane channel, and the resultant change in membrane permeability allows leakage of intracellular components . In its liposomal formulation, this compound is integrated with the liposome membranes, forming a non-covalent complex between mycosamine (positively charged) and distearoylphosphatidylglycerol (DMPG) (negatively charged), as well as hydrophobic interactions .
Temporal Effects in Laboratory Settings
The effects of this compound over time in laboratory settings have been observed to be potent and dose-dependent in a large number of animal models . The long terminal half-life and retention in tissues suggest that single or intermittent dosing regimens are feasible .
Dosage Effects in Animal Models
The effects of this compound vary with different dosages in animal models . It has been observed that the drug exhibits potent, dose-dependent activity in a large number of animal models .
Metabolic Pathways
This compound is involved in one carbon pool by folate, which was confirmed as the key metabolic pathway related to this compound biosynthesis . Overexpression of genes involved in this pathway, such as methionine synthase (metH), has been shown to increase this compound production .
Transport and Distribution
This compound is generally considered to be transported and distributed within cells and tissues via its integration with liposome membranes in its liposomal formulation . This forms a non-covalent complex between mycosamine (positively charged) and distearoylphosphatidylglycerol (DMPG) (negatively charged), as well as hydrophobic interactions .
Subcellular Localization
The subcellular localization of this compound and its effects on its activity or function have been observed in the cell walls of mature cells and the plasma membranes of the daughter cells at the budding stage . It has been shown to interfere with the formation of functional cell walls of such young cells .
Preparation Methods
The synthesis of Amphotericin A involves a series of complex reactions. The primary synthetic route includes the fermentation of Streptomyces nodosus, a soil bacterium, which produces Amphotericin B. This compound is then derived from Amphotericin B through a series of chemical modifications . Industrial production methods typically involve large-scale fermentation processes followed by purification steps to isolate the desired compound .
Chemical Reactions Analysis
Amphotericin A undergoes various chemical reactions, including oxidation, reduction, and substitution reactions. Common reagents used in these reactions include strong oxidizing agents like potassium permanganate and reducing agents such as sodium borohydride . The major products formed from these reactions depend on the specific conditions and reagents used. For example, oxidation of this compound can lead to the formation of various oxidized derivatives, while reduction can yield reduced forms of the compound .
Scientific Research Applications
Amphotericin A has a wide range of scientific research applications. In chemistry, it is used as a model compound for studying polyene antifungal agents. In biology and medicine, it is extensively studied for its antifungal properties and its potential use in treating fungal infections . Additionally, this compound is used in the pharmaceutical industry for the development of new antifungal drugs .
Comparison with Similar Compounds
Amphotericin A is similar to other polyene antifungal compounds such as Amphotericin B and Nystatin. it is unique in its specific binding affinity and spectrum of activity . Amphotericin B, for example, is more widely used in clinical settings due to its broader antifungal spectrum, while Nystatin is primarily used for topical applications . The unique properties of this compound make it a valuable compound for specific research and therapeutic applications .
Properties
IUPAC Name |
(1R,3S,5R,6R,9R,11R,15S,16R,17R,18S,19E,21E,25E,27E,29E,31E,33R,35S,36R,37S)-33-[(2R,3S,4S,5S,6R)-4-amino-3,5-dihydroxy-6-methyloxan-2-yl]oxy-1,3,5,6,9,11,17,37-octahydroxy-15,16,18-trimethyl-13-oxo-14,39-dioxabicyclo[33.3.1]nonatriaconta-19,21,25,27,29,31-hexaene-36-carboxylic acid | |
---|---|---|
Source | PubChem | |
URL | https://pubchem.ncbi.nlm.nih.gov | |
Description | Data deposited in or computed by PubChem | |
InChI |
InChI=1S/C47H75NO17/c1-27-17-15-13-11-9-7-5-6-8-10-12-14-16-18-34(64-46-44(58)41(48)43(57)30(4)63-46)24-38-40(45(59)60)37(54)26-47(61,65-38)25-33(51)22-36(53)35(52)20-19-31(49)21-32(50)23-39(55)62-29(3)28(2)42(27)56/h5-6,8,10-18,27-38,40-44,46,49-54,56-58,61H,7,9,19-26,48H2,1-4H3,(H,59,60)/b6-5+,10-8+,13-11+,14-12+,17-15+,18-16+/t27-,28-,29-,30+,31+,32+,33-,34-,35+,36+,37-,38-,40+,41-,42+,43+,44-,46-,47+/m0/s1 | |
Source | PubChem | |
URL | https://pubchem.ncbi.nlm.nih.gov | |
Description | Data deposited in or computed by PubChem | |
InChI Key |
QGGFZZLFKABGNL-MOISJGEISA-N | |
Source | PubChem | |
URL | https://pubchem.ncbi.nlm.nih.gov | |
Description | Data deposited in or computed by PubChem | |
Canonical SMILES |
CC1C=CC=CCCC=CC=CC=CC=CC(CC2C(C(CC(O2)(CC(CC(C(CCC(CC(CC(=O)OC(C(C1O)C)C)O)O)O)O)O)O)O)C(=O)O)OC3C(C(C(C(O3)C)O)N)O | |
Source | PubChem | |
URL | https://pubchem.ncbi.nlm.nih.gov | |
Description | Data deposited in or computed by PubChem | |
Isomeric SMILES |
C[C@H]1/C=C/C=C/CC/C=C/C=C/C=C/C=C/[C@@H](C[C@H]2[C@@H]([C@H](C[C@](O2)(C[C@H](C[C@H]([C@@H](CC[C@H](C[C@H](CC(=O)O[C@H]([C@@H]([C@@H]1O)C)C)O)O)O)O)O)O)O)C(=O)O)O[C@H]3[C@H]([C@H]([C@@H]([C@H](O3)C)O)N)O | |
Source | PubChem | |
URL | https://pubchem.ncbi.nlm.nih.gov | |
Description | Data deposited in or computed by PubChem | |
Molecular Formula |
C47H75NO17 | |
Source | PubChem | |
URL | https://pubchem.ncbi.nlm.nih.gov | |
Description | Data deposited in or computed by PubChem | |
Molecular Weight |
926.1 g/mol | |
Source | PubChem | |
URL | https://pubchem.ncbi.nlm.nih.gov | |
Description | Data deposited in or computed by PubChem | |
CAS No. |
1405-32-9 | |
Record name | Amphotericin A | |
Source | CAS Common Chemistry | |
URL | https://commonchemistry.cas.org/detail?cas_rn=1405-32-9 | |
Description | CAS Common Chemistry is an open community resource for accessing chemical information. Nearly 500,000 chemical substances from CAS REGISTRY cover areas of community interest, including common and frequently regulated chemicals, and those relevant to high school and undergraduate chemistry classes. This chemical information, curated by our expert scientists, is provided in alignment with our mission as a division of the American Chemical Society. | |
Explanation | The data from CAS Common Chemistry is provided under a CC-BY-NC 4.0 license, unless otherwise stated. | |
Record name | Amphotericin A | |
Source | ChemIDplus | |
URL | https://pubchem.ncbi.nlm.nih.gov/substance/?source=chemidplus&sourceid=0001405329 | |
Description | ChemIDplus is a free, web search system that provides access to the structure and nomenclature authority files used for the identification of chemical substances cited in National Library of Medicine (NLM) databases, including the TOXNET system. | |
Record name | AMPHOTERICIN A | |
Source | FDA Global Substance Registration System (GSRS) | |
URL | https://gsrs.ncats.nih.gov/ginas/app/beta/substances/6KQ75HL677 | |
Description | The FDA Global Substance Registration System (GSRS) enables the efficient and accurate exchange of information on what substances are in regulated products. Instead of relying on names, which vary across regulatory domains, countries, and regions, the GSRS knowledge base makes it possible for substances to be defined by standardized, scientific descriptions. | |
Explanation | Unless otherwise noted, the contents of the FDA website (www.fda.gov), both text and graphics, are not copyrighted. They are in the public domain and may be republished, reprinted and otherwise used freely by anyone without the need to obtain permission from FDA. Credit to the U.S. Food and Drug Administration as the source is appreciated but not required. | |
Disclaimer and Information on In-Vitro Research Products
Please be aware that all articles and product information presented on BenchChem are intended solely for informational purposes. The products available for purchase on BenchChem are specifically designed for in-vitro studies, which are conducted outside of living organisms. In-vitro studies, derived from the Latin term "in glass," involve experiments performed in controlled laboratory settings using cells or tissues. It is important to note that these products are not categorized as medicines or drugs, and they have not received approval from the FDA for the prevention, treatment, or cure of any medical condition, ailment, or disease. We must emphasize that any form of bodily introduction of these products into humans or animals is strictly prohibited by law. It is essential to adhere to these guidelines to ensure compliance with legal and ethical standards in research and experimentation.