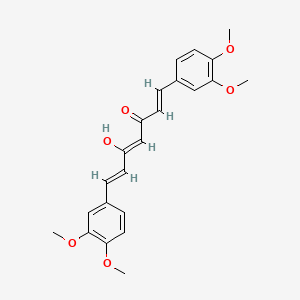
Dimethylcurcumin
Overview
Description
Dimethoxycurcumin is a synthetic analogue of curcumin, a natural compound found in turmericDimethoxycurcumin is characterized by the presence of methoxy groups on the aromatic rings, which enhance its metabolic stability compared to curcumin . This compound has gained attention due to its potential therapeutic properties, including anti-inflammatory, antioxidant, and anticancer activities .
Mechanism of Action
Target of Action
ASC-J9, also known as Dimethylcurcumin, is primarily targeted towards the Androgen Receptor (AR) . The AR is a type of nuclear receptor that is activated by binding any of the androgenic hormones, including testosterone and dihydrotestosterone .
Mode of Action
Instead, it appears to act as a Selective Androgen Receptor Degradation Enhancer (SARD) . This means that ASC-J9 enhances the degradation of certain subpopulations of the AR, particularly those present in the prostate gland .
Biochemical Pathways
ASC-J9 has been found to suppress the phosphorylation/activation of STAT3 and the expression of CCL2, with little influence on the PIAS3 expression in AR-negative PC3 cells . This suggests that ASC-J9 could also modulate STAT3 phosphorylation/activation and CCL2 expression through an AR-independent pathway .
Pharmacokinetics
One of the major challenges with the use of curcumin, from which ASC-J9 is derived, is its poor solubility and low metabolic stability, which hinder its bioavailability . Asc-j9, being a lipophilic compound, maintains its anticancer potency and has greatly improved systemic bioavailability .
Result of Action
ASC-J9 effectively suppresses the growth and invasion of castration-resistant prostate cancer cells . It achieves this by degrading both full-length and splice variant androgen receptors .
Biochemical Analysis
Biochemical Properties
Dimethylcurcumin plays a significant role in biochemical reactions by interacting with various biomolecules. It primarily targets the androgen receptor (AR), promoting its degradation rather than merely inhibiting its activity . This interaction is crucial in reducing the proliferation of androgen-dependent cells, such as those found in prostate cancer . Additionally, this compound has been shown to interact with the glutamate-cysteine ligase catalytic subunit, leading to increased expression of activating transcription factor 3 (ATF3), which suppresses cancer cell proliferation and invasion .
Cellular Effects
This compound exerts profound effects on various cell types and cellular processes. In prostate cancer cells, it significantly inhibits cell proliferation and invasion by degrading androgen receptors . This compound also influences cell signaling pathways, such as the ATF3-PTK2 signaling pathway, which is involved in tumor suppression . Furthermore, this compound affects gene expression by modulating the levels of specific transcription factors and enzymes, thereby altering cellular metabolism and function .
Molecular Mechanism
The molecular mechanism of this compound involves its role as a selective androgen receptor degrader. Unlike traditional antiandrogens that block the receptor, this compound promotes the degradation of androgen receptors, particularly in prostate cells . This degradation is facilitated by binding interactions that target specific subpopulations of androgen receptors, leading to their breakdown and subsequent reduction in androgen-dependent cell growth . Additionally, this compound modulates apoptosis signals, further contributing to its anticancer effects .
Temporal Effects in Laboratory Settings
In laboratory settings, the effects of this compound have been observed to change over time. Studies have shown that its stability and degradation can influence its long-term efficacy. For instance, the half maximum inhibitory concentrations (IC50) of this compound increase with cell confluency, indicating that its effectiveness may vary depending on the cellular environment . Long-term studies have demonstrated that this compound maintains its AR degradation activity over extended periods, making it a promising candidate for sustained therapeutic applications .
Dosage Effects in Animal Models
The effects of this compound vary with different dosages in animal models. In studies involving nude mice with xenografted prostate cancer cells, this compound significantly decreased the development of metastatic tumors at a dosage of 75 mg/kg, administered intraperitoneally three times per week for three weeks . Higher doses have been associated with increased efficacy, but potential toxic or adverse effects at very high doses have not been extensively documented .
Metabolic Pathways
This compound is involved in several metabolic pathways, primarily through its interaction with androgen receptors and other enzymes. It modulates the expression of glutamate-cysteine ligase catalytic subunit, which plays a role in cellular antioxidant defense . This interaction leads to changes in metabolic flux and metabolite levels, contributing to its anticancer properties . Additionally, this compound’s lipophilic nature enhances its bioavailability and systemic distribution .
Transport and Distribution
Within cells and tissues, this compound is transported and distributed through interactions with specific transporters and binding proteins. Its lipophilic properties facilitate its accumulation in lipid-rich environments, enhancing its therapeutic efficacy . Encapsulation in nanoparticles has been explored to improve its targeted delivery and reduce systemic side effects . These strategies have shown promise in enhancing the anticancer activity of this compound by ensuring its efficient distribution to target cells .
Subcellular Localization
This compound’s subcellular localization is critical for its activity and function. It predominantly localizes to the cytoplasm, where it interacts with androgen receptors and other target proteins . Post-translational modifications and targeting signals direct this compound to specific cellular compartments, ensuring its effective engagement with target biomolecules . This precise localization is essential for its role in degrading androgen receptors and modulating cellular processes .
Preparation Methods
Synthetic Routes and Reaction Conditions: Dimethoxycurcumin can be synthesized through various synthetic routes. One common method involves the condensation of vanillin with acetone in the presence of a base, followed by methylation of the resulting product . The reaction conditions typically include the use of a strong base such as sodium hydroxide or potassium hydroxide, and the methylation step is often carried out using methyl iodide or dimethyl sulfate .
Industrial Production Methods: Industrial production of dimethoxycurcumin involves similar synthetic routes but on a larger scale. The process is optimized for higher yields and purity, often involving advanced purification techniques such as recrystallization and chromatography . The scalability of the synthesis process makes it feasible for commercial production and application in various industries.
Chemical Reactions Analysis
Types of Reactions: Dimethoxycurcumin undergoes several types of chemical reactions, including:
Common Reagents and Conditions:
Oxidation: Common oxidizing agents include hydrogen peroxide, potassium permanganate, and chromium trioxide.
Reduction: Reducing agents such as sodium borohydride and lithium aluminum hydride are commonly used.
Substitution: Substitution reactions often involve reagents like halogens, alkylating agents, and nucleophiles.
Major Products: The major products formed from these reactions include various oxidized, reduced, and substituted derivatives of dimethoxycurcumin, each with unique chemical and biological properties .
Scientific Research Applications
Comparison with Similar Compounds
Dimethoxycurcumin is compared with other curcumin analogues such as:
Curcumin: The parent compound with well-documented biological activities but limited by poor bioavailability and metabolic stability.
Demethoxycurcumin: Lacks one methoxy group and exhibits similar but slightly different biological activities.
Bisdemethoxycurcumin: Lacks both methoxy groups and has distinct chemical and biological properties.
Tetrahydrocurcumin: A reduced derivative with enhanced stability and different biological activities.
Uniqueness of Dimethoxycurcumin: Dimethoxycurcumin stands out due to its enhanced metabolic stability and bioavailability compared to curcumin. Its unique chemical structure allows for better interaction with biological targets, making it a promising candidate for therapeutic applications.
Properties
IUPAC Name |
1,7-bis(3,4-dimethoxyphenyl)-5-hydroxyhepta-1,4,6-trien-3-one | |
---|---|---|
Details | Computed by LexiChem 2.6.6 (PubChem release 2019.06.18) | |
Source | PubChem | |
URL | https://pubchem.ncbi.nlm.nih.gov | |
Description | Data deposited in or computed by PubChem | |
InChI |
InChI=1S/C23H24O6/c1-26-20-11-7-16(13-22(20)28-3)5-9-18(24)15-19(25)10-6-17-8-12-21(27-2)23(14-17)29-4/h5-15,24H,1-4H3 | |
Details | Computed by InChI 1.0.5 (PubChem release 2019.06.18) | |
Source | PubChem | |
URL | https://pubchem.ncbi.nlm.nih.gov | |
Description | Data deposited in or computed by PubChem | |
InChI Key |
ZMGUKFHHNQMKJI-UHFFFAOYSA-N | |
Details | Computed by InChI 1.0.5 (PubChem release 2019.06.18) | |
Source | PubChem | |
URL | https://pubchem.ncbi.nlm.nih.gov | |
Description | Data deposited in or computed by PubChem | |
Canonical SMILES |
COC1=C(C=C(C=C1)C=CC(=CC(=O)C=CC2=CC(=C(C=C2)OC)OC)O)OC | |
Details | Computed by OEChem 2.1.5 (PubChem release 2019.06.18) | |
Source | PubChem | |
URL | https://pubchem.ncbi.nlm.nih.gov | |
Description | Data deposited in or computed by PubChem | |
Molecular Formula |
C23H24O6 | |
Details | Computed by PubChem 2.1 (PubChem release 2019.06.18) | |
Source | PubChem | |
URL | https://pubchem.ncbi.nlm.nih.gov | |
Description | Data deposited in or computed by PubChem | |
Molecular Weight |
396.4 g/mol | |
Details | Computed by PubChem 2.1 (PubChem release 2021.05.07) | |
Source | PubChem | |
URL | https://pubchem.ncbi.nlm.nih.gov | |
Description | Data deposited in or computed by PubChem | |
Retrosynthesis Analysis
AI-Powered Synthesis Planning: Our tool employs the Template_relevance Pistachio, Template_relevance Bkms_metabolic, Template_relevance Pistachio_ringbreaker, Template_relevance Reaxys, Template_relevance Reaxys_biocatalysis model, leveraging a vast database of chemical reactions to predict feasible synthetic routes.
One-Step Synthesis Focus: Specifically designed for one-step synthesis, it provides concise and direct routes for your target compounds, streamlining the synthesis process.
Accurate Predictions: Utilizing the extensive PISTACHIO, BKMS_METABOLIC, PISTACHIO_RINGBREAKER, REAXYS, REAXYS_BIOCATALYSIS database, our tool offers high-accuracy predictions, reflecting the latest in chemical research and data.
Strategy Settings
Precursor scoring | Relevance Heuristic |
---|---|
Min. plausibility | 0.01 |
Model | Template_relevance |
Template Set | Pistachio/Bkms_metabolic/Pistachio_ringbreaker/Reaxys/Reaxys_biocatalysis |
Top-N result to add to graph | 6 |
Feasible Synthetic Routes
Q1: What is the primary target of ASC-J9 and how does it exert its effects?
A1: ASC-J9 primarily targets the androgen receptor (AR) [, , , , , , , , , , , , , ]. Unlike traditional anti-androgens that compete with androgens for AR binding, ASC-J9 enhances AR degradation [, , ]. This leads to the downregulation of AR signaling pathways, impacting various downstream processes involved in prostate cancer, BPH, and other AR-related diseases [, , , , , , , , , , , , , ].
Q2: How does ASC-J9's mechanism of action differ from that of traditional anti-androgens like Casodex or MDV3100?
A2: Unlike Casodex or MDV3100, which antagonize AR by competing with androgens, ASC-J9 enhances AR degradation, leading to a more profound suppression of AR signaling [, ]. Studies have shown that Casodex and MDV3100 can actually promote prostate cancer metastasis through alternative pathways, whereas ASC-J9 demonstrates anti-metastatic properties [, , ].
Q3: What are the downstream effects of ASC-J9 mediated AR degradation?
A3: ASC-J9's action on AR leads to a cascade of downstream effects, including:
- Inhibition of prostate cancer cell growth and metastasis [, , , , , , ]
- Suppression of prostate stromal cell growth in BPH [, ]
- Reduction of bladder cancer progression []
- Modulation of inflammatory responses in various diseases [, , , , , ]
- Accelerated cutaneous wound healing []
- Enhanced T-cell reconstitution and bone marrow transplant grafting efficacy []
Q4: What is the molecular formula, weight, and spectroscopic data of ASC-J9?
A4: ASC-J9 (Dimethylcurcumin) has the following characteristics:
Q5: Is there information available on the material compatibility and stability of ASC-J9 under various conditions?
A5: While the provided research focuses on the biological effects of ASC-J9, limited information is available regarding its material compatibility and stability under various conditions beyond its solubility in different solvents for experimental use [, , ]. Further research is needed to explore these aspects.
Q6: Does ASC-J9 possess any catalytic properties or have applications in catalysis?
A6: The provided research primarily focuses on the biological activities and therapeutic potential of ASC-J9. There is no mention of catalytic properties or applications in the context of these studies.
Q7: Has computational chemistry been used to study ASC-J9?
A7: The provided research focuses mainly on in vitro and in vivo studies. While it mentions the synthesis and structure-activity relationships of ASC-J9 and its analogues, it doesn't delve into specific computational chemistry studies or QSAR models [, ].
Q8: What is known about the stability and formulation strategies for ASC-J9?
A8: While the provided research doesn't specifically address the stability of ASC-J9 under various conditions, it highlights the compound's poor bioavailability when administered alone []. Research suggests that consuming curcuminoids as part of a turmeric matrix, such as in fresh or powdered turmeric, significantly enhances bioavailability compared to isolated curcumin powder []. This suggests that the natural matrix and co-presence of other turmeric compounds might offer stabilizing and bioavailability-enhancing effects.
Q9: Are there any specific formulation strategies being explored to improve ASC-J9's stability, solubility, or bioavailability?
A9: Although the research doesn't mention specific formulation strategies for ASC-J9, one study describes the synthesis of ethylene-carbonate-linked L-valine derivatives of 4,4-Dimethylcurcumin to improve its solubility and potentially enhance cellular uptake and anticancer activity []. This approach might be applicable to ASC-J9 as well. Another study used an electrospun scaffold loaded with ASC-J9 for wound healing, suggesting a potential delivery system for localized application [].
Disclaimer and Information on In-Vitro Research Products
Please be aware that all articles and product information presented on BenchChem are intended solely for informational purposes. The products available for purchase on BenchChem are specifically designed for in-vitro studies, which are conducted outside of living organisms. In-vitro studies, derived from the Latin term "in glass," involve experiments performed in controlled laboratory settings using cells or tissues. It is important to note that these products are not categorized as medicines or drugs, and they have not received approval from the FDA for the prevention, treatment, or cure of any medical condition, ailment, or disease. We must emphasize that any form of bodily introduction of these products into humans or animals is strictly prohibited by law. It is essential to adhere to these guidelines to ensure compliance with legal and ethical standards in research and experimentation.