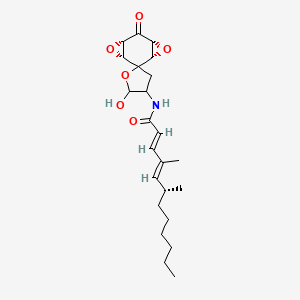
Aranorosin
Overview
Description
Aranorosin is a novel antibiotic compound isolated from the fermentation broth of the fungal strain Pseudoarachniotus roseus . It exhibits a wide spectrum of biological activity, particularly as an antifungal agent . The compound has garnered significant interest due to its unique chemical structure and potent biological properties .
Mechanism of Action
Target of Action
Aranorosin, also known as Aranorosine, primarily targets the bifunctional enzyme AAC(6’)/APH(2") . This enzyme is responsible for the resistance of Methicillin-resistant Staphylococcus aureus (MRSA) to the antibiotic arbekacin . This compound inhibits this enzyme, thereby circumventing the resistance mechanism .
Mode of Action
This compound interacts with its target, the bifunctional enzyme AAC(6’)/APH(2"), by inhibiting its function . This enzyme catalyzes both the phosphorylation and acetylation of aminoglycosides, which is a key mechanism of arbekacin resistance in MRSA . By inhibiting this enzyme, this compound prevents the inactivation of arbekacin, thus restoring the antibiotic’s effectiveness against MRSA .
Biochemical Pathways
It is known that this compound’s inhibition of the aac(6’)/aph(2") enzyme disrupts the normal function of this enzyme, which is a key component of the arbekacin resistance mechanism in mrsa
Pharmacokinetics
Like all drugs, these properties would significantly impact this compound’s bioavailability and overall effectiveness
Result of Action
The primary result of this compound’s action is the circumvention of arbekacin resistance in MRSA . By inhibiting the AAC(6’)/APH(2") enzyme, this compound prevents the inactivation of arbekacin, allowing the antibiotic to effectively combat MRSA infections . This has significant implications for the treatment of MRSA, particularly in cases where the bacteria have developed resistance to other antibiotics.
Biochemical Analysis
Biochemical Properties
Aranorosin plays a crucial role in biochemical reactions, particularly in its interaction with various enzymes and proteins. One notable interaction is with the bifunctional enzyme AAC(6’)/APH(2″), which is involved in antibiotic resistance mechanisms in methicillin-resistant Staphylococcus aureus (MRSA) . This compound inhibits this enzyme, thereby circumventing resistance and restoring the efficacy of antibiotics like arbekacin . Additionally, this compound has been shown to interact with Bcl-2, a protein that regulates apoptosis, inhibiting its anti-apoptotic functions . These interactions highlight the compound’s potential in combating antibiotic resistance and modulating cell death pathways.
Cellular Effects
This compound exerts significant effects on various cell types and cellular processes. In MRSA, this compound inhibits the bifunctional enzyme AAC(6’)/APH(2″), leading to the circumvention of antibiotic resistance . This inhibition restores the effectiveness of antibiotics, allowing for successful treatment of resistant bacterial strains. Furthermore, this compound has been found to inhibit the anti-apoptotic functions of Bcl-2, promoting apoptosis in cancer cells . This dual action on bacterial and cancer cells underscores the compound’s potential as a versatile therapeutic agent.
Molecular Mechanism
The molecular mechanism of this compound involves its binding interactions with specific biomolecules. By inhibiting the bifunctional enzyme AAC(6’)/APH(2″), this compound prevents the phosphorylation and acetylation of aminoglycosides, which are crucial for antibiotic resistance in MRSA . This inhibition restores the susceptibility of MRSA to antibiotics like arbekacin. Additionally, this compound’s interaction with Bcl-2 disrupts its anti-apoptotic functions, leading to increased apoptosis in cancer cells . These molecular interactions highlight the compound’s potential in overcoming antibiotic resistance and promoting cell death in cancer cells.
Temporal Effects in Laboratory Settings
In laboratory settings, the effects of this compound have been observed to change over time. The compound’s stability and degradation have been studied extensively, revealing that it remains stable under specific conditions . Long-term studies have shown that this compound maintains its efficacy in inhibiting the bifunctional enzyme AAC(6’)/APH(2″) and promoting apoptosis in cancer cells . These findings suggest that this compound can be a reliable therapeutic agent with sustained effects over time.
Dosage Effects in Animal Models
The effects of this compound vary with different dosages in animal models. Studies have shown that lower doses of this compound effectively inhibit the bifunctional enzyme AAC(6’)/APH(2″) in MRSA, restoring antibiotic susceptibility . Higher doses may lead to toxic or adverse effects, highlighting the importance of determining the optimal dosage for therapeutic applications . These findings underscore the need for careful dosage optimization in future studies.
Metabolic Pathways
This compound is involved in specific metabolic pathways, interacting with enzymes and cofactors that influence its activity. The compound’s interaction with the bifunctional enzyme AAC(6’)/APH(2″) is a key aspect of its metabolic pathway, as it inhibits the enzyme’s phosphorylation and acetylation activities . This inhibition disrupts the metabolic processes that contribute to antibiotic resistance in MRSA. Additionally, this compound’s interaction with Bcl-2 affects cellular metabolism by promoting apoptosis in cancer cells . These metabolic interactions highlight the compound’s potential in modulating key biochemical pathways.
Transport and Distribution
This compound is transported and distributed within cells and tissues through specific mechanisms. The compound’s interaction with transporters and binding proteins influences its localization and accumulation . Studies have shown that this compound is effectively transported to target cells, where it exerts its inhibitory effects on the bifunctional enzyme AAC(6’)/APH(2″) and Bcl-2 . This targeted distribution enhances the compound’s therapeutic potential by ensuring its delivery to specific cellular compartments.
Subcellular Localization
The subcellular localization of this compound plays a crucial role in its activity and function. The compound is directed to specific compartments within cells, where it interacts with target biomolecules . This compound’s localization to the cytoplasm allows it to inhibit the bifunctional enzyme AAC(6’)/APH(2″) and disrupt antibiotic resistance mechanisms in MRSA . Additionally, its localization to the mitochondria and other organelles enables it to interact with Bcl-2 and promote apoptosis in cancer cells . These subcellular interactions highlight the importance of this compound’s precise localization in mediating its therapeutic effects.
Preparation Methods
Aranorosin is primarily produced through microbial fermentation. The fungal strain Pseudoarachniotus roseus is cultured in specific media under controlled conditions to produce this compound . The fermentation process involves maintaining the fungal strain on Sabouraud’s glucose agar and YpSs agar, followed by transferring the culture to a seed medium containing soluble starch, soybean meal, glucose, calcium carbonate, sodium chloride, yeast extract, and corn steep liquor . The production medium is then inoculated with the seed culture and incubated on a rotary shaker at 26°C for 72 hours .
Chemical Reactions Analysis
Aranorosin undergoes various chemical reactions, including oxidation, reduction, and substitution . For instance, it reacts with lithium chloride at room temperature in tetrahydrofuran, forming a variety of chlorinated products under mild conditions . The compound contains a novel l-oxaspiro[4,5]decane ring system, which is crucial for its biological activity . Common reagents used in these reactions include chloride salts and oxidizing agents .
Scientific Research Applications
Aranorosin has several scientific research applications, particularly in the fields of chemistry, biology, and medicine. It has been studied for its antifungal properties and its ability to circumvent antibiotic resistance in methicillin-resistant Staphylococcus aureus (MRSA) by inhibiting the bifunctional enzyme AAC(6’)/APH(2") . Additionally, this compound has shown growth inhibitory activity against human triple-negative breast cancer cells . Its unique chemical structure and biological activity make it a valuable compound for developing new therapeutic agents .
Comparison with Similar Compounds
Aranorosin is structurally related to other compounds produced by the same fungal strain, such as aranochlor A and aranochlor B . These compounds share a similar core structure but differ in specific functional groups. For instance, aranochlor A and aranochlor B contain chlorine atoms and lack an epoxide group, which is present in this compound . Additionally, this compound can be compared to gymnastatin and dankastatin alkaloids, which are also produced by fungal strains and exhibit similar biological activities . this compound’s unique l-oxaspiro[4,5]decane ring system and its potent antifungal properties distinguish it from these related compounds .
Properties
IUPAC Name |
(2E,4E,6R)-N-[(1S,3R,5S,7R)-2'-hydroxy-6-oxospiro[4,8-dioxatricyclo[5.1.0.03,5]octane-2,5'-oxolane]-3'-yl]-4,6-dimethyldodeca-2,4-dienamide | |
---|---|---|
Source | PubChem | |
URL | https://pubchem.ncbi.nlm.nih.gov | |
Description | Data deposited in or computed by PubChem | |
InChI |
InChI=1S/C23H33NO6/c1-4-5-6-7-8-13(2)11-14(3)9-10-16(25)24-15-12-23(30-22(15)27)20-18(28-20)17(26)19-21(23)29-19/h9-11,13,15,18-22,27H,4-8,12H2,1-3H3,(H,24,25)/b10-9+,14-11+/t13-,15?,18-,19+,20-,21+,22?,23?/m1/s1 | |
Source | PubChem | |
URL | https://pubchem.ncbi.nlm.nih.gov | |
Description | Data deposited in or computed by PubChem | |
InChI Key |
JHTWWPWUODMKEO-PHJKOLFRSA-N | |
Source | PubChem | |
URL | https://pubchem.ncbi.nlm.nih.gov | |
Description | Data deposited in or computed by PubChem | |
Canonical SMILES |
CCCCCCC(C)C=C(C)C=CC(=O)NC1CC2(C3C(O3)C(=O)C4C2O4)OC1O | |
Source | PubChem | |
URL | https://pubchem.ncbi.nlm.nih.gov | |
Description | Data deposited in or computed by PubChem | |
Isomeric SMILES |
CCCCCC[C@@H](C)/C=C(\C)/C=C/C(=O)NC1CC2([C@@H]3[C@@H](O3)C(=O)[C@@H]4[C@H]2O4)OC1O | |
Source | PubChem | |
URL | https://pubchem.ncbi.nlm.nih.gov | |
Description | Data deposited in or computed by PubChem | |
Molecular Formula |
C23H33NO6 | |
Source | PubChem | |
URL | https://pubchem.ncbi.nlm.nih.gov | |
Description | Data deposited in or computed by PubChem | |
Molecular Weight |
419.5 g/mol | |
Source | PubChem | |
URL | https://pubchem.ncbi.nlm.nih.gov | |
Description | Data deposited in or computed by PubChem | |
CAS No. |
117184-53-9 | |
Record name | Aranorosin | |
Source | ChemIDplus | |
URL | https://pubchem.ncbi.nlm.nih.gov/substance/?source=chemidplus&sourceid=0117184539 | |
Description | ChemIDplus is a free, web search system that provides access to the structure and nomenclature authority files used for the identification of chemical substances cited in National Library of Medicine (NLM) databases, including the TOXNET system. | |
Retrosynthesis Analysis
AI-Powered Synthesis Planning: Our tool employs the Template_relevance Pistachio, Template_relevance Bkms_metabolic, Template_relevance Pistachio_ringbreaker, Template_relevance Reaxys, Template_relevance Reaxys_biocatalysis model, leveraging a vast database of chemical reactions to predict feasible synthetic routes.
One-Step Synthesis Focus: Specifically designed for one-step synthesis, it provides concise and direct routes for your target compounds, streamlining the synthesis process.
Accurate Predictions: Utilizing the extensive PISTACHIO, BKMS_METABOLIC, PISTACHIO_RINGBREAKER, REAXYS, REAXYS_BIOCATALYSIS database, our tool offers high-accuracy predictions, reflecting the latest in chemical research and data.
Strategy Settings
Precursor scoring | Relevance Heuristic |
---|---|
Min. plausibility | 0.01 |
Model | Template_relevance |
Template Set | Pistachio/Bkms_metabolic/Pistachio_ringbreaker/Reaxys/Reaxys_biocatalysis |
Top-N result to add to graph | 6 |
Feasible Synthetic Routes
Q1: What is the molecular formula and weight of aranorosin?
A1: this compound has the molecular formula C23H33NO6 and a molecular weight of 419.5 g/mol. []
Q2: What spectroscopic data is available for characterizing this compound?
A2: this compound's structure has been elucidated using various spectroscopic techniques, including Nuclear Magnetic Resonance (1H NMR and 13C NMR), Circular Dichroism (CD) spectroscopy, and mass spectrometry. These techniques provide detailed information about the compound's connectivity, stereochemistry, and functional groups. [, , ]
Q3: What are the known biological activities of this compound?
A3: this compound exhibits a broad spectrum of biological activities, most notably antifungal and antitumor effects. It displays activity against various fungi and has shown promising results in inhibiting the growth of cancer cells. [, ]
Q4: How does this compound exert its antitumor activity?
A4: While the exact mechanism of action remains partially unclear, research suggests this compound might interfere with the anti-apoptotic function of the Bcl-2 protein. By doing so, it could potentially promote cancer cell death. Further research is needed to fully understand the molecular interactions involved. []
Q5: Does this compound exhibit activity against drug-resistant bacteria?
A5: Interestingly, this compound has demonstrated the ability to circumvent arbekacin resistance in Methicillin-resistant Staphylococcus aureus (MRSA). This effect is attributed to its inhibition of the bifunctional enzyme AAC(6')/APH(2"), which is involved in aminoglycoside resistance. This finding highlights this compound's potential to address the growing concern of antibiotic resistance. []
Q6: What is known about the Structure-Activity Relationship (SAR) of this compound and its analogs?
A6: Studies investigating the SAR of this compound have primarily focused on modifying the fatty acid side chain. These modifications aim to improve its antifungal activity. Research indicates that altering the length and functional groups within the side chain can significantly impact the compound's potency and spectrum of activity. [, ]
Q7: What synthetic approaches have been employed to produce this compound and its analogs?
A7: Several total syntheses of this compound have been achieved, employing various strategies. Key steps often include oxidative cyclization of tyrosine derivatives, stereoselective epoxidations, and coupling reactions to introduce the fatty acid side chain. These synthetic routes allow for the preparation of this compound and facilitate the development of novel analogs with potentially improved biological properties. [, , , , , , ]
Q8: Have any simplified analogs of this compound been synthesized?
A8: Yes, researchers have synthesized a model system for the this compound nucleus. This simplified structure, 2-hydroxy-6,7;9,10-cis,cis-diepoxy-1-oxaspiro[4.5]decan-8-one, retains the core spirocyclic framework and diepoxide functionality found in the natural product. X-ray crystallography confirmed its stereochemistry to match that of this compound. Such models are valuable tools for studying the compound's reactivity and exploring structure-activity relationships. []
Q9: What is known about the biosynthesis of this compound?
A9: While the complete biosynthetic pathway of this compound remains to be fully elucidated, it is suggested to originate from tyrosine. Intriguingly, research suggests potential biosynthetic connections between this compound and other structurally complex natural products, such as the gymnastatin and dankastatin alkaloids, which are also produced by Gymnascella sp. fungi. This observation opens avenues for exploring the shared biosynthetic pathways and potential enzymatic transformations involved. []
Disclaimer and Information on In-Vitro Research Products
Please be aware that all articles and product information presented on BenchChem are intended solely for informational purposes. The products available for purchase on BenchChem are specifically designed for in-vitro studies, which are conducted outside of living organisms. In-vitro studies, derived from the Latin term "in glass," involve experiments performed in controlled laboratory settings using cells or tissues. It is important to note that these products are not categorized as medicines or drugs, and they have not received approval from the FDA for the prevention, treatment, or cure of any medical condition, ailment, or disease. We must emphasize that any form of bodily introduction of these products into humans or animals is strictly prohibited by law. It is essential to adhere to these guidelines to ensure compliance with legal and ethical standards in research and experimentation.