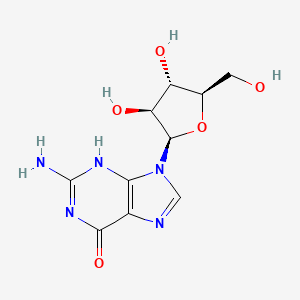
9-beta-d-Arabinofuranosylguanine
Overview
Description
9-beta-d-Arabinofuranosylguanine (ara-G) is a deoxyguanosine analog . It was synthesized in 1964, but due to its difficult chemical synthesis and poor solubility properties, it was not until 1995 that a prodrug of ara-G, nelarabine, entered clinical trials .
Synthesis Analysis
The synthesis of ara-G from 1-(beta-D-arabinofuranosyl)cytosine (ara-C) and guanine, guanosine, or 2’-deoxyguanosine (dG) by glutaraldehyde-treated Escherichia coli BM-11 cells has been described . The concentration of phosphate ions, molar ratio of substrates, and pH of the reaction medium are factors affecting product yield .
Chemical Reactions Analysis
Ara-G needs to be phosphorylated inside the cell for pharmacological activity . In the first and rate-limiting phosphorylation step, ara-G is a substrate of both the mitochondrial deoxyguanosine kinase and the cytosolic deoxycytidine kinase . Once phosphorylated to its triphosphate derivative, ara-GTP acts as a structural analog of deoxyribonucleotide 5’-triphosphate and is thereby incorporated into DNA .
Scientific Research Applications
Cancer Therapy
9-beta-d-Arabinofuranosylguanine (ara-G) is a deoxyguanosine analog that has shown promising results in cancer therapy . It needs to be phosphorylated inside the cell for pharmacological activity . Once phosphorylated to its triphosphate derivative, ara-GTP, it acts as a structural analog of deoxyribonucleotide 5’-triphosphate and is thereby incorporated into DNA . The accumulation of the triphosphate form of ara-G results in inhibition of DNA synthesis and subsequent cell death .
Treatment of T-cell Malignancies
Ara-G has shown higher toxicity in T than B lymphoblasts . Nelarabine, a prodrug of ara-G, has shown promising effect not only in patients with T-cell malignancies but also in patients with B-cell malignancies . Nelarabine has now earned fast track status from the US Food and Drug Administration for the treatment of T-cell acute lymphoblastic leukemia and lymphoblastic lymphoma .
Synthesis using Escherichia coli
Ara-G can be synthesized from 1-(beta-d-arabinofuranosyl)cytosine (ara-C) and guanine, guanosine or 2’-deoxyguanosine (dG) by glutaraldehyde-treated Escherichia coli BM-11 cells . The concentration of phosphate ions, molar ratio of substrates and pH of the reaction medium are factors affecting product yield . Under optimum conditions, ara-G was produced in the reaction mixture in a yield of 63%-65% based on dG as the best source of guanine base .
Mechanism of Action
Target of Action
9-beta-d-Arabinofuranosylguanine (ara-G) is a deoxyguanosine analog . Its primary targets are the mitochondrial deoxyguanosine kinase and the cytosolic deoxycytidine kinase . These enzymes play a crucial role in the phosphorylation of ara-G, which is necessary for its pharmacological activity .
Mode of Action
Ara-G needs to be phosphorylated inside the cell for pharmacological activity . In the first and rate-limiting phosphorylation step, ara-G is a substrate of both the mitochondrial deoxyguanosine kinase and the cytosolic deoxycytidine kinase . Once phosphorylated to its triphosphate derivative, ara-GTP, it acts as a structural analog of deoxyribonucleotide 5?-triphosphate and is thereby incorporated into DNA .
Biochemical Pathways
The accumulation of the triphosphate form of ara-G results in inhibition of DNA synthesis . This process affects the DNA replication pathway, leading to subsequent cell death . Ara-G is selectively toxic to cultured T-lymphoblasts due to their ability to accumulate higher levels of the cytotoxic metabolite, ara-GTP, relative to B- and null lymphoblastoid cells .
Pharmacokinetics
Ara-G is a water-soluble prodrug . It is phosphorylated within leukemic cells to form ara-G triphosphate (ara-GTP), which acts to terminate DNA chain elongation, resulting in cell death . The peak concentration of ara-G was found to be 115 μmol/L, and it had a half-life of 3.4 hours .
Result of Action
The incorporation of ara-G into DNA inhibits DNA synthesis, leading to cell death .
Action Environment
The concentration of phosphate ions, molar ratio of substrates, and pH of the reaction medium are factors affecting the yield of ara-G . Under optimum conditions, ara-G was produced in the reaction mixture in a yield of 63%-65% based on dG as the best source of guanine base . The yield of isolated ara-G was 48%-53% .
Safety and Hazards
Future Directions
Nelarabine, a prodrug of ara-G, has earned fast track status from the US Food and Drug Administration for the treatment of T-cell acute lymphoblastic leukemia and lymphoblastic lymphoma in those who have not responded to or whose disease has progressed during treatment with at least two standard regimens . Nelarabine is an interesting new anticancer agent that might be used as a standard treatment in the future .
properties
IUPAC Name |
2-amino-9-[(2R,3S,4S,5R)-3,4-dihydroxy-5-(hydroxymethyl)oxolan-2-yl]-1H-purin-6-one | |
---|---|---|
Source | PubChem | |
URL | https://pubchem.ncbi.nlm.nih.gov | |
Description | Data deposited in or computed by PubChem | |
InChI |
InChI=1S/C10H13N5O5/c11-10-13-7-4(8(19)14-10)12-2-15(7)9-6(18)5(17)3(1-16)20-9/h2-3,5-6,9,16-18H,1H2,(H3,11,13,14,19)/t3-,5-,6+,9-/m1/s1 | |
Source | PubChem | |
URL | https://pubchem.ncbi.nlm.nih.gov | |
Description | Data deposited in or computed by PubChem | |
InChI Key |
NYHBQMYGNKIUIF-FJFJXFQQSA-N | |
Source | PubChem | |
URL | https://pubchem.ncbi.nlm.nih.gov | |
Description | Data deposited in or computed by PubChem | |
Canonical SMILES |
C1=NC2=C(N1C3C(C(C(O3)CO)O)O)N=C(NC2=O)N | |
Source | PubChem | |
URL | https://pubchem.ncbi.nlm.nih.gov | |
Description | Data deposited in or computed by PubChem | |
Isomeric SMILES |
C1=NC2=C(N1[C@H]3[C@H]([C@@H]([C@H](O3)CO)O)O)N=C(NC2=O)N | |
Source | PubChem | |
URL | https://pubchem.ncbi.nlm.nih.gov | |
Description | Data deposited in or computed by PubChem | |
Molecular Formula |
C10H13N5O5 | |
Source | PubChem | |
URL | https://pubchem.ncbi.nlm.nih.gov | |
Description | Data deposited in or computed by PubChem | |
DSSTOX Substance ID |
DTXSID301019130 | |
Record name | 9-Arabinofuranosylguanine | |
Source | EPA DSSTox | |
URL | https://comptox.epa.gov/dashboard/DTXSID301019130 | |
Description | DSSTox provides a high quality public chemistry resource for supporting improved predictive toxicology. | |
Molecular Weight |
283.24 g/mol | |
Source | PubChem | |
URL | https://pubchem.ncbi.nlm.nih.gov | |
Description | Data deposited in or computed by PubChem | |
Product Name |
9-beta-d-Arabinofuranosylguanine | |
CAS RN |
38819-10-2 | |
Record name | 9-β-D-Arabinofuranosylguanine | |
Source | CAS Common Chemistry | |
URL | https://commonchemistry.cas.org/detail?cas_rn=38819-10-2 | |
Description | CAS Common Chemistry is an open community resource for accessing chemical information. Nearly 500,000 chemical substances from CAS REGISTRY cover areas of community interest, including common and frequently regulated chemicals, and those relevant to high school and undergraduate chemistry classes. This chemical information, curated by our expert scientists, is provided in alignment with our mission as a division of the American Chemical Society. | |
Explanation | The data from CAS Common Chemistry is provided under a CC-BY-NC 4.0 license, unless otherwise stated. | |
Record name | 9-Arabinofuranosylguanine | |
Source | ChemIDplus | |
URL | https://pubchem.ncbi.nlm.nih.gov/substance/?source=chemidplus&sourceid=0038819102 | |
Description | ChemIDplus is a free, web search system that provides access to the structure and nomenclature authority files used for the identification of chemical substances cited in National Library of Medicine (NLM) databases, including the TOXNET system. | |
Record name | 9-Arabinofuranosylguanine | |
Source | EPA DSSTox | |
URL | https://comptox.epa.gov/dashboard/DTXSID301019130 | |
Description | DSSTox provides a high quality public chemistry resource for supporting improved predictive toxicology. | |
Record name | 9 BETA ARABINOFURANOSYLGUANINE | |
Source | European Chemicals Agency (ECHA) | |
URL | https://echa.europa.eu/information-on-chemicals | |
Description | The European Chemicals Agency (ECHA) is an agency of the European Union which is the driving force among regulatory authorities in implementing the EU's groundbreaking chemicals legislation for the benefit of human health and the environment as well as for innovation and competitiveness. | |
Explanation | Use of the information, documents and data from the ECHA website is subject to the terms and conditions of this Legal Notice, and subject to other binding limitations provided for under applicable law, the information, documents and data made available on the ECHA website may be reproduced, distributed and/or used, totally or in part, for non-commercial purposes provided that ECHA is acknowledged as the source: "Source: European Chemicals Agency, http://echa.europa.eu/". Such acknowledgement must be included in each copy of the material. ECHA permits and encourages organisations and individuals to create links to the ECHA website under the following cumulative conditions: Links can only be made to webpages that provide a link to the Legal Notice page. | |
Record name | ARAGUANOSINE | |
Source | FDA Global Substance Registration System (GSRS) | |
URL | https://gsrs.ncats.nih.gov/ginas/app/beta/substances/0Z99WX0GPF | |
Description | The FDA Global Substance Registration System (GSRS) enables the efficient and accurate exchange of information on what substances are in regulated products. Instead of relying on names, which vary across regulatory domains, countries, and regions, the GSRS knowledge base makes it possible for substances to be defined by standardized, scientific descriptions. | |
Explanation | Unless otherwise noted, the contents of the FDA website (www.fda.gov), both text and graphics, are not copyrighted. They are in the public domain and may be republished, reprinted and otherwise used freely by anyone without the need to obtain permission from FDA. Credit to the U.S. Food and Drug Administration as the source is appreciated but not required. | |
Retrosynthesis Analysis
AI-Powered Synthesis Planning: Our tool employs the Template_relevance Pistachio, Template_relevance Bkms_metabolic, Template_relevance Pistachio_ringbreaker, Template_relevance Reaxys, Template_relevance Reaxys_biocatalysis model, leveraging a vast database of chemical reactions to predict feasible synthetic routes.
One-Step Synthesis Focus: Specifically designed for one-step synthesis, it provides concise and direct routes for your target compounds, streamlining the synthesis process.
Accurate Predictions: Utilizing the extensive PISTACHIO, BKMS_METABOLIC, PISTACHIO_RINGBREAKER, REAXYS, REAXYS_BIOCATALYSIS database, our tool offers high-accuracy predictions, reflecting the latest in chemical research and data.
Strategy Settings
Precursor scoring | Relevance Heuristic |
---|---|
Min. plausibility | 0.01 |
Model | Template_relevance |
Template Set | Pistachio/Bkms_metabolic/Pistachio_ringbreaker/Reaxys/Reaxys_biocatalysis |
Top-N result to add to graph | 6 |
Feasible Synthetic Routes
Disclaimer and Information on In-Vitro Research Products
Please be aware that all articles and product information presented on BenchChem are intended solely for informational purposes. The products available for purchase on BenchChem are specifically designed for in-vitro studies, which are conducted outside of living organisms. In-vitro studies, derived from the Latin term "in glass," involve experiments performed in controlled laboratory settings using cells or tissues. It is important to note that these products are not categorized as medicines or drugs, and they have not received approval from the FDA for the prevention, treatment, or cure of any medical condition, ailment, or disease. We must emphasize that any form of bodily introduction of these products into humans or animals is strictly prohibited by law. It is essential to adhere to these guidelines to ensure compliance with legal and ethical standards in research and experimentation.